Malformation artério-veineuse cérébrale
Rédigé par Atos Alves de Sousa, MD, PhD / Lucas Alverne Freitas de Albuquerque, MD / Marcos Dellaretti, MD, PhD / Belo Horizonte, MG, Brazil
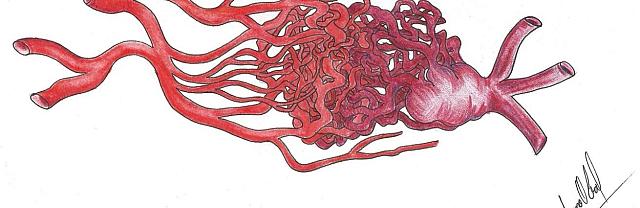
I. Definition
McCormick in 1966 and Russell and Rubenstein described four types of vascular malformations, and this is now accepted as the current nomenclature. [62-64] Cerebrovascular malformations are classified according to their histopathologic features as : arteriovenous malformation (AVMs), venous angioma, cavernous malformation, and capillary telangiectasia. The focus of this chapter is on true AVMs. A possible fifth category is a direct fistula, or arteriovenous fistula (AVF). These conditions are regarded as acquired lesions involving single or multiple dilated arterioles that connect directly to a vein without a nidus. They are high-flow, high-pressure lesions that have a low incidence of hemorrhage (examples include vein of Galen aneurysmal malformation, dural AVF, and carotid cavernous fistula). [62-64]
AVMs are vascular anomalies that may occur in any region of the brain. They are composed of a nidus with feeding arteries, and draining veins that form an anomalous mass of blood vessels in the pia matter, with direct arteriovenous shunts and a poor or absent capillary bed, and consequently a high-flow shunt that predisposes to arterialization of veins, vascular recruitment, and gliosis of brain tissue adjacent to the lesion. [30, 48]
Originally it was thought that the nidus lacked a true capillary bed. However, Sato et al. [90] analyzing the relationships between perinidal vessels and the nidus in 22 resected specimens from patients with cerebral AVMs, observed that all nidus were accompanied by a perinidal dilated capillary network that was connected not only to the nidus, feeding arteries, and draining veins, via arterioles and venules, but also to normal capillaries, arterioles, and venules. There is the hypothesis that these capillaries may contribute to the intraoperative and postoperative bleeding, growth, and even recurrence of surgically treated cerebral AVMs, as these perinidal capillaries may fuse to become part of the nidus [90].
Nidal vessels are structurally ambiguous resembling both arteries and veins, with high risk of intracranial hemorrhage, which is the main and the most feared manifestation of AVMs, followed by epileptic seizures. AVM-high-flow shunts cause great dilation of the drainage veins that can result in a mass effect. At the same time, they can provoke a reduction of the perfusion pressure in the adjacent brain, that being known as “brain vascular steal”, which is an important mechanism in the pathogenesis of focal neurological deficits. [18, 54, 56, 58]
++++
II. History
Colby et al. [13] performed in 2012 a review of the history of AVMs, from their very beginning until today. We highlight chronologically some of the most fundamental aspects of this long history [13] :
– Mid-1700s - John Hunter (1728–1793) described the clinical characteristics of extracranial AVMs.
– 1863 - Rudolf Virchow (1821–1902) described many of the common intracranial vascular pathologic entities, including AVMs.
– 1889 - Davide Giordano (1864–1954) - the first report of a palliative treatment of a true cerebral AVM by ligation of a left parietal feeding artery.
– 1889 – Jules-Émile Péan (1830 – 1898) - the first complete excision of a cerebral AVM was made by the famous French surgeon J-E Péan. [112]
– 1914 - Vilhelm Magnus (1871–1929) was probably the first to treat cerebral AVMs with radiation using conventional fractionated radiation.
– 1927 - Antonio Caetano de Abreu Freire Egas Moniz (1874–1955) performed the first successful cerebral angiogram by injecting contrast into the carotid artery of patients. A major advance for the diagnosis and understanding of AVMs.
– 1928 - Walter Dandy (1886–1946) and Harvey Cushing (1869–1939) with Percival Bailey (1892–1973) - independently reported a series of AVMs treated before the introduction of angiography, with primarily catastrophic results in both series.
– 1932 - Herbert Olivecrona (1891–1980) was the second to successfully remove an AVM. He introduced the technique of ligating superficial feeding vessels and then working in a circumferential fashion until the deep portion of the AVM was dissected and separated from the brain. The AVM draining veins were ligated as a final step.
– 1932 to 1957 - Gazi Yasargil (born 1925) compiled information from the literature on 500 AVMs : operative mortality for “small” AVMs was 5% and for “moderate-size” AVMs was 10%.
– 1950s - Leonard Malis (1919–1995) devised and constructed bipolar coagulation forceps.
– 1951 - Lars Leksell (1907–1986) described the radiosurgery, using a cyclotron.
– Early-1960s - Luessenhop and William Spence (1908–1992) - developed largely, endovascular techniques conceptualizing the blockage of hypertrophied, abnormal feeding vessels, by direct puncture of target vessels to introduce catheters and embolic material.
– 1960s - The introduction of the operative microscope and the resulting development of microsurgical instruments and microsurgical techniques
– 1966 - George Perret and Hiro Nishioka – initiated the study of AVM natural history reporting an analysis of 545 cases of cerebral AVMs and fistulae with a hemorrhage rate of 1.5%per year.
– 1968 - Leksell introduced the first Gamma Knife using cobalt sources.
– 1969 - Yasargil – published the first microsurgical AVM series, and his results were excellent.
– 1971 - Atkinson Morley’s Hospital in England – first clinical CT scan on a patient.
– 1974 – Fedor Serbinenko (1928–2002) – in the endovascular field, described the balloon catheterization and occlusion of intracranial vessels.
– 1976 - Charles Kerber - in the endovascular field, described a calibrated leak balloon system for flow-directed catheter guidance and administration of contrast and cyanoacrylate embolic material.
– 1977 - Downstate Medical Center in New York - first clinical MRI on a patient.
– 1986 - Robert Spetzler and Neil Martin developed the important AVM classification scheme based on lesion size, venous drainage, and eloquence of involved brain.
– 1990s - development of functional MRI and diffusion tensor imaging to map eloquent areas of brain and their relationship to the lesion.
– 1991 - Osvaldo Betti and colleagues introduced the radiosurgery using LINAC (linear accelerator)
– End-2010s - Intraoperative fluorescence video angiography using indocyanine green (ICG).
++++
III. Natural history
The mean AVM risk of bleeding varies in literature from 2 to 4% per year [15, 20, 31, 36, 78, 79, 101, 108]. The mortality risk is 1% per year [20, 78, 79, 101, 108]. After a first bleeding, the risk of re-bleeding rises to 6 to 18% in the first year, then goes back to the previous risk in the years that follow [20, 59, 103]. However, Ondra et al. [79] and Crawford et al. [13] demonstrated that 6 months after a bleed, the risk of hemorrhage is the same for patients with prior hemorrhage and for patients who have never had a hemorrhage.
The lifetime risk of bleeding is between 17 to 90% [78, 101]. Lehecka et al. [50] proposed that the percentage risk to have a fatal bleeding from an untreated AVM during a life time may be roughly estimated as : (90 - age in years)%.
After each AVM bleed there is a mortality rate of 5 to 15% and an additional 20% to 30% risk of serious morbidity [26, 89]. Some series describes a bad functional evolution in even 30 to 56% of patients [5, 33, 73].
Not all AVMs present the same risk of bleeding, and some factors are related to a greater risk. Hernesniemi et al. [31] in a study of 238 patients with a mean follow-up period of 13.5 years found an annual risk of hemorrhage from AVMs of 2.4%. They indicated as risk factors predicting subsequent AVM hemorrhage in univariate analysis : young age, previous rupture, deep and infratentorial locations, and exclusively deep venous drainage. In addition, they indicated as independent risk factors according to multivariate models : previous rupture, large AVM size, and infratentorial and deep locations.
Other risks factors indicated mentioned in literature [20] :
– Intranidal aneurysm : 15% of all AVMs are associated to aneurysms that may be located in the feeding arteries (flow aneurysms), in arteries not related to the irrigation of the AVM or in the nidus (intranidal). Redekop et al. [87] reviewed 632 cases of AVMs diagnosed through angiography and found an incidence of 5.5% of intranidal aneurysms, 11.2% of flow aneurysms and 0.8% of aneurysms not related to the malformation. It is still unknown if there is a difference among those locations when reporting hemorrhages. Some authors [6] recommend to first treat the aneurysm in asymptomatic patients. They say that after resecting the AVM there is a supposed greater risk of aneurysm rupture due to a temporary increase in the pressure of the feeding artery. Other authors consider that asymptomatic flow aneurysms do not need to be treated because after the excision of the AVM these aneurysms spontaneously disappear.
– Ventricular or paraventricular AVM ;
– Stenosis of the draining vein (the risk of bleeding should be directly proportional to the degree of stenosis) ;
– Number of draining veins (the risk of bleeding is inversely proportional to the number of draining veins, probably due to the increase of flow and turbulence in the case of a smaller number of draining channels [66], being of a higher risk in cases of a single draining vein) ;
Small AVMs are also listed as a risk factor according to some authors. This is a very controversial aspect, so Hernesniemi et al. [31] in his series pointed out that large AVMs have a higher risk of bleeding. We believe that there is an important confounding aspect, because the only clinical manifestation of the small AVM is related to bleeding, so one may have the wrong impression that they are more prone to bled.
AVMs that never bled without bleeding risk factors may present a lower risk of bleeding, such as 1% a year whereas those with bleeding risk factors that also never bled present a higher risk of bleeding of 8% a year. On the other hand, in the case of AVMs that already bled and that present bleeding risk factors, the re-bleeding rate in the first year can reach 35% [20, 99].
Autopsy studies conclude that only 12% of all AVMs became symptomatic in a lifetime, what may be related to the lack of data on the history of bleeding in such type of study [60, 61].
The “A Randomized Trial of Un-ruptured Brain AVMs” (ARUBA) is a trial that aims to assess the outcomes of patients with un-ruptured AVMs randomized to medical management alone (ie, pharmacological therapy for neurological symptoms as needed) versus medical management with interventional therapy (ie, neurosurgery, embolisation, or stereotactic radiotherapy, alone or in combination) [70, 71]. The primary outcome was the time to the composite endpoint of death or symptomatic stroke ; and the primary analysis was by the intention to treat. [71]
The original plan was to randomize 800 patients with un-ruptured brain AVMs and determine whether or not the risks of treatment outweighed the risks of conservative management over 5 years. However, the randomization was stopped prematurely by the data safety monitoring board with a mean follow up of 33 months and 223 patients (114 assigned to interventional therapy and 109 to medical management) because of the superiority of the medical management group. [71]
The demographic characteristics were similar between the two groups. However the risk of death or stroke was significantly lower in the medical management group (11 patients – 10.1%) than in the interventional therapy group (35 patients – 30.7%). [71] However, as the findings of this study did not consider the degree of AVM, it should not be used to guide management in these patients.
The ARUBA trial in its 33 months of follow up concluded that medical management alone is superior to medical management with interventional therapy for the prevention of death or stroke in patients with un-ruptured brain arteriovenous malformations. [71] They will continue its observational phase to establish whether the disparities will persist over an additional 5 years of follow-up.
Stapf et al. [100] and Starke et al. [101] analyzed preview data from ARUBA and observed that Spetzler Martin grade I and II demonstrates similar outcomes in medical and surgical group, but significantly worse outcomes in grade III and IV AVM in the surgical group. Besides this some of ARUBA’s limitations are highlighted by Starke et al [101] and include the short follow up period ; small sample size ; the study is not powered to evaluate the treatment effect by an individual modality and the great heterogeneity of the treatment and experience in each center. Besides, these and many other methodological inconsistences and bias were predicted by Cockroft et al. [12] and put in to check ARUBA’s value [69].
++++
IV. Epidemiology
AVMs are the most common cerebrovascular malformations. However, it is hard to assess their real incidence and prevalence as many are asymptomatic, and so are underestimated. Based on imaging tests, symptomatic AVM incidence ranges from 0.89 to 4 per 100,000 people/year [2, 5, 8, 10, 24, 38, 98]. Unfortunately it is impossible to estimate the real incidence of asymptomatic AVMs. Based on autopsy studies, the prevalence is rather discrepant, ranging from five to 513 per 100,000 people [14, 37, 61]. There is an increase in the diagnosis of incidental AVMs with the advances in neuroimaging.
AVMs typically present before the age of 40 (20-40 years), mean age being 33 years [20, 103]. There is a low prevalence in women, however without statistical significance [23].
Although AVMs are rare lesions and the bleedings due to AVMs correspond to only 2% of all cerebrovascular strokes [20], they represent the primary cause of intracerebral hemorrhage (ICH) in young adults (16.7%), followed by aneurysms (15.5%) [10, 73].
++++
V. Pathophysiological principles of the pathological process
1. Etiology
The true pathogenesis (when lesion formation is initiated, how it typically progresses, or how progression is impacted by environmental risk factors) of AVMs is unknown and the currently available animal models of cerebrovascular malformation still have limitations. [13, 48]
Many authors believe that cerebrovascular malformation arises during embryonic development and two main hypotheses for AVM pathogenesis include : embryonic agenesis of the capillary system and the retention of a primordial connection between arteries and veins. [30] However these hypotheses are not proved, and little direct evidence supports these ideas.
As proposed by Leblanc et al. [48] some (perhaps most) cerebrovascular malformations arise post-natally and into adulthood. They believe that studying cerebrovascular malformation genes function in adult angiogenesis and vascular repair and how their expression is affected by environmental perturbations will help in the understanding of AVM etiology. As said in epidemiology, AVMs typically present at a mean age of 33 years, and not in childhood as should be expected in congenital lesions [20, 103]. In addition, there is clear evidence that AVMs are dynamic and biologically active lesions rather than static congenital vascular abnormalities, with growth or regress, changes in their structure and form again later in life [17, 30, 65].
AVMs can occur either sporadically or in the context of genetic syndromes. The primary one associated with cerebral AVM is hereditary hemorrhagic telangiectasia (HHT) [48]. Nevertheless, AVMs may be associated to other well-defined genetic diseases : Osler-Weber-Rendu disease, telangiectasia ataxia, Wyburn-Mason syndrome, and Sturge-Weber syndrome [42, 44, 67].
More than 900 genes are known to be involved in the pathogenesis of AVMs and their molecular characterization, pattern definition, and family heritage relationships are a challenge [67]. Some genes highlighted by Leblanc et al. [48] are Endoglin (ENG), Activin-like kinase receptor 1 (ACVRL1 ; ALK-1) and SMAD4. They propose that HHT Types 1 and 2 result from loss-of-function mutations in one copy of the Endoglin (ENG) and Activin-like kinase receptor 1 (ACVRL1 ; ALK-1) genes, respectively. ALK-1 variants may also be associated with risk for sporadic AVM [82]. The SMAD4, were described in some cases of combined juvenile polyposis and HHT syndrome [22].
About the formation of AVMs there is a more current hypothesis of the genetic “two-hit” mechanism, in which an inherited mutation in one copy of a cerebrovascular malformation gene is followed by a somatic mutation in a second copy [48, 55]. As observed by Leblanc et al. [48], alternatively, the second “hit” could be environmental in the form of a localized physiological or pathological perturbation. There is an interaction between genetic factors related to vasculogenesis and hemodynamic factors. The development of AVMs is related to genetics, a number of growth factors and extracellular proteins that may inhibit or stimulate their growth and reshaping, besides their structural and hemodynamic features.
++++
2. Physiology
2.1. Pressure in feeding arteries
Smaller malformations (≤ 3 cm), because of greater vascular resistance, are supposed to be associated with a higher pressure in feeding arteries and, thus, have a greater chance probability of bleeding [77, 94]. However, this is a controversial hypothesis, because normally the small AVMs do not present epilepsy or flow steal phenomenon, and the only presentation is bleeding, and so a bias of presentation.
2.2. Venous contribution
According to some authors, anomalies in the venous components are predecessors of AVMs [68]. It is believed that, during the embryogenesis, anomalies in the cerebrovascular venous system may occur, such as venous occlusion, stenosis, or agenesis [67]. As time passes, factors such as venous hypertension end up transforming such anomalies into brain AVMs. Chronic venous hypertension raises intraluminal pressure, which may lead to the reduction of tissue perfusion and, then, ischemia [67], which releases chemical mediators that favor angiogenesis, besides diapedesis, that also favor angiogenesis if in excess by means of factors such as vascular endothelial growth factor (VEGF) [109]. Angiogenesis leads to the formation of arteriovenous fistulas, which cause an increase in the pressure of the venous bed, resulting in a vicious cycle that provokes the growth of AVMs.
Another theory is that the obstruction of venous drainage may cause the opening of pre-existing arteriovenous connections [109], thus leading to the formation of an AVM.
Some venous features are more highly subjected to bleeding, such as exclusively deep venous drainage, venous stenosis, and venous reflow [74]. On the other hand, arterial stenosis and arterial ectasia are not associated to a higher risk of bleeding.
2.3. Regulation of blood flow
AVMs are associated to the loss of blood flow regulation (impaired autoregulation), but it is not known if they cause it or result from it.
The “normal perfusion pressure breakthrough theory”, by Spetzler et al [97], advocates the possibility that auto-regulation is altered due to the AVM.
Other authors [68] say that auto-regulation changes are the cause for the growth and remodeling of AVMs, and may happen in a multi-factorial manner :
– Arterial : high trans-nidal flow and arterial hypertension ;
– Venous : hypertension, thrombosis or stenosis ;
– Others : vascular lesion, abnormal endothelial signs, micro-shunt formation.
2.4. AVM Structure
The structure of an AVM may range from really simple (Figure 1a), with a single compact nidus, one feeding artery and one or more draining veins, to very complex (Figure 1b), with multiple feeding arteries and draining veins, resulting in multiple nidus compartments, that can be contiguous or separated by a small amount of brain tissue, functional or not [68, 75, 113].
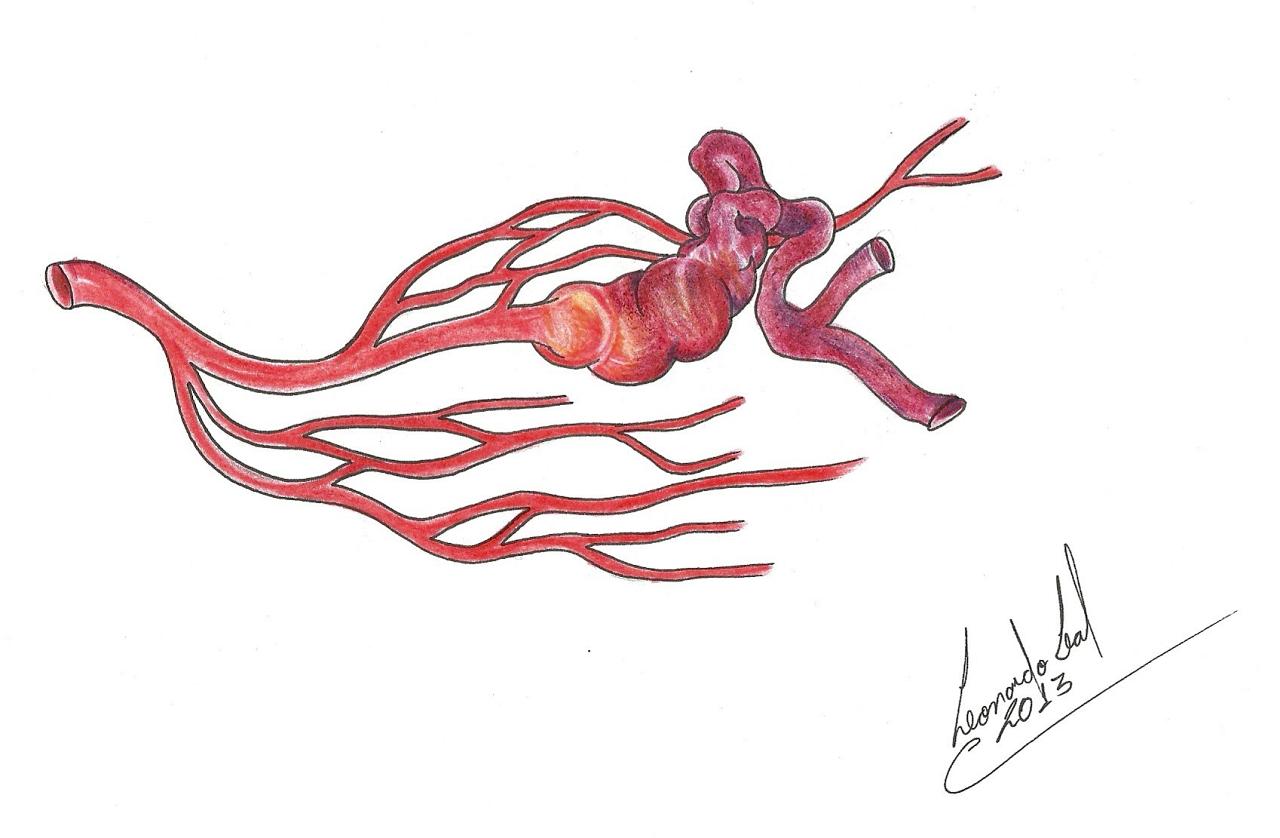
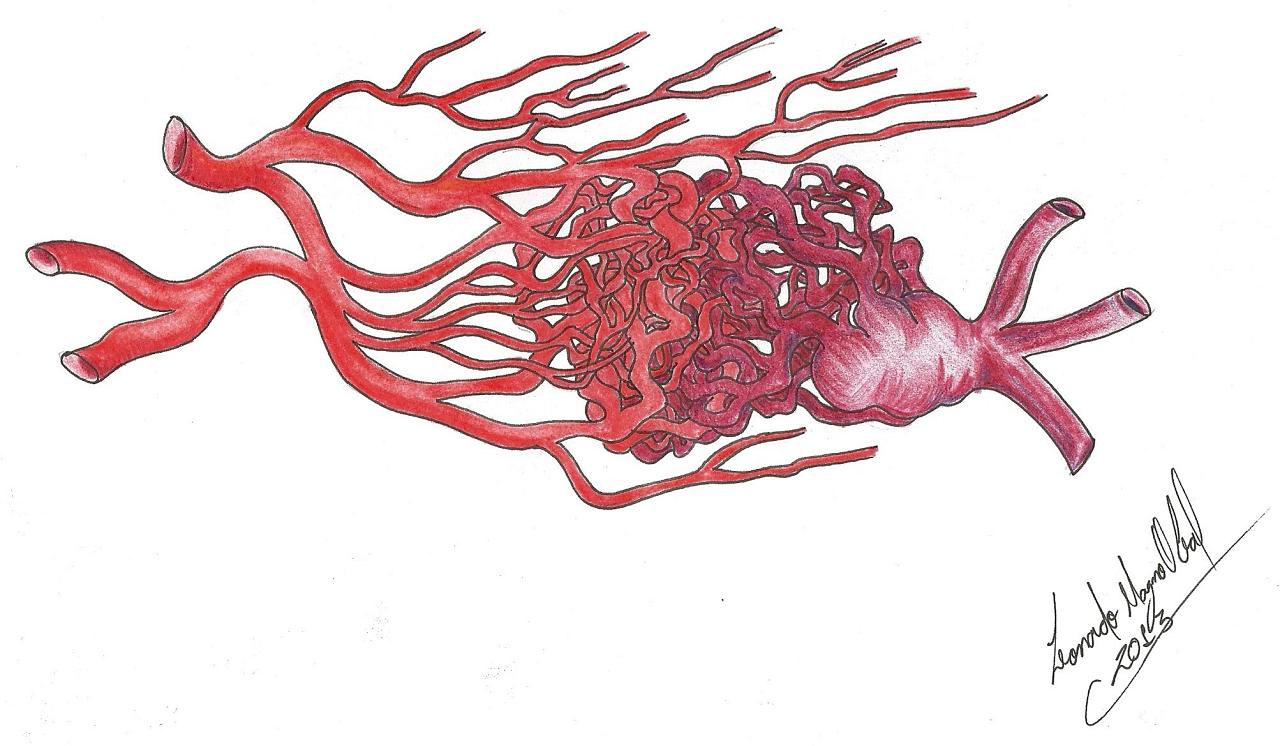
“Hidden compartments” can be present, showing as non-filled during angiography but in the follow-up may be filled and thus suggest AVM growth. Such compartments can be filled even after apparently complete AVM resection, thus suggesting a de novo AVM. ”Hidden compartments” can usually be identified through super-selective digital angiography [29].
The nidus can be :
– Single : compact or diffuse (with one compartment) ;
– Multiple : compact or diffuse (with more than one compartment).
2.5. Perinidal vessels
The terms “reserve nidus” and “perinidal dilated capillary network” refer to abnormal perinidal vessels that can be seen through angiography and that may later become part of the nidus [68, 90]. Due to the fact that perinidal vessels are connected to the nidus, it is believed that they are related to postoperative re-bleeding or de novo AVMs.
The terms “modja-modja” and “shaggy hair” are used to describe a hypervascular perinidal network. As they tend to bleed after nidal resection, it is advisable to coagulate these abnormal vessels, if present, during surgery and to induce hypotension in the postoperative course so as to lower the chances of re-bleeding and edema.
2.6. Flow steal
Brain AVMs are characterized by low resistance within the nidus secondary to the lack of an inter-posing capillary bed, thus depriving surrounding parenchyma of blood flow [115]. Flow steal caused by high flow arteriovenous shunt may be the cause of symptoms in some AVMs.
However there are controversies about its in vivo existence due to the disagreement regarding the several techniques that have been used to research it [68].
++++
VI. Diagnosis
1. Clinical features
AVMs can be asymptomatic or symptomatic, but the great development and advances in imaging methods have made it possible to diagnose incidental AVMs earlier nowadays.
In cases of symptomatic AVMs, the main clinical manifestations are : intracranial hemorrhage, seizures, and headache. Approximately 50% of symptomatic patients (42 to 72% in the literature) present intracranial hemorrhage, the first event happening between 20 and 40 years of age, in both male and female [20]. AVM rupture may result in subarachnoid, intracerebral or intraventricular hemorrhage.
Epilepsy comes in second place, occurring in 34% of the cases, mainly simple partial seizures and complex partial seizures. Headaches is the first symptom in 31% of the cases, without any specific feature. Focal neurological signs are present in 40% of the patients [58]. Other manifestations should also be considered, such as focal motor and sensitive deficits, speech disorder, visual deficits, and so forth.
Part of the symptoms are related to the angio architecture : as patients with afferent meningeal arteries are more prone to headache ; as previously mentioned, more risk of hemorrhage in previous rupture, large AVM size, infratentorial and deep locations, intranidal aneurysm, etc. ; progressive neurologic deficit by flow steal phenomenon in large AVMs.
The clinical presentation of the Vein of Galen malformation varies according to age. Neonates tend to present with high-output cardiac failure, pulmonary hypertension, and, in more severe cases, multiorgan system failure. Infants commonly present with hydrocephalus, seizures, or neurocognitive delay. Older children and adults usually present with headaches or intracranial hemorrhage.
++++
2. Imaging findings
Radiological examinations are essential for the diagnosis of AVMs.
– Computed tomography (CT) is an excellent exam in emergency situations so as to identify acute hemorrhage (Figure 2). It is also useful to evaluate AVM calcification. In the CT, AVMs do not have any specific presentation, showing density similar to normal vessels, therefore a little denser than cerebral tissue and with high contrast enhancement, which helps to define the borders of the lesion. It can be difficult to detect an AVM through CT mainly when the nidus is smaller than 1 cm in diameter.
– Magnetic Resonance Imaging (MRI) is an excellent exam to diagnose AVMs allowing a three dimensional visualization of the lesion and thus helping to plan the surgical and radiosurgical treatment. For being constituted by countless vessels, AVMs show in MRI as flow voids in T1 and T2 weighted images and are enhanced by contrasts (Figure 3).
MRI also detects recent or old hematomas ; and the Fluid-Attenuated Inversion-Recovery (FLAIR) sequence can show perinidal ischemic alterations, vasogenic edema or gliosis. A functional MRI (MRf) is also possible in order to relate the lesion to eloquent areas (motor, visual and speech).
– Magnetic Resonance angiography (MRA) is useful in seeing the anatomy of the AVM in detail, showing details of its vessels. However it is not as good as digital angiography in the hemodynamic evaluation and for anatomic details, which are very important in treatment planning.
– Dynamic contrast-enhanced MRA is a promising non-invasive technique for studying AVMs. Images are acquired at multiple time points based on separation of the arterial, capillary, and venous phases of contrast passage to identify abnormal filling of the feeding arteries, nidus, and draining vessels of the AVM during the arterial phase. [72, 76, 88] Therefore the images produced are not static as in CTA or MRA, and may provide information about the hemodynamics of the AVMs. It produces high enough spatial and temporal resolution to enable us to visualize in detail the vascular architecture of the AVM. However, the detailed evaluation by conventional angiography is still the gold standard, because the current dynamic contrast-enhanced MRA does not yet have enough spatial and temporal resolution. [76]
Other benefits in relation to Computed Tomography Angiography (CTA) are the avoidance of radiation exposure, use of iodinated contrast material and in after intervention follow up, because clips and endovascular cast produces significant image artifacts in CTA. [72, 76]
– Computed Tomography Angiography (CTA) provides even more detailed information on the AVM vascularization than MRI, but less information on its relationship with brain parenchyma.
– Whole-brain CT angiography and perfusion combines dynamic 3-dimensional CT angiography of the brain with whole-brain CT perfusion imaging. [72, 80] It provides excellent spatial resolution, allows recognition of important AVM properties and may exhibit different pathologic phenomena, such as the arterial steal phenomenon and venous congestion. [72]
– Digital Subtraction Angiography (DSA) is the gold standard exam in the study of AVMs (Figure 4). It is necessary to analyze internal and external carotid arteries and vertebral arteries to learn about [87] :
– The location and the real size of the nidus ;
– The AVM arterial supply, including the deep and transventricular feeders ;
– Type of arteriovenous shunt (high versus low flow) ;
– Venous drainage pattern ;
– Presence of aneurysms in the feeding arteries or intranidal aneurysms ;
– Presence of venous stenosis, obstructions or abnormalities in the venous drainage ;
– Time distribution of arterial, parenchymatous and venous phases. The nidus is prematurely filled in the arterial phase due to the direct shunt from arteries to veins, making a type of bypass and making drainage veins tortuous and ectasic in response to the high blood flow and higher pressure.
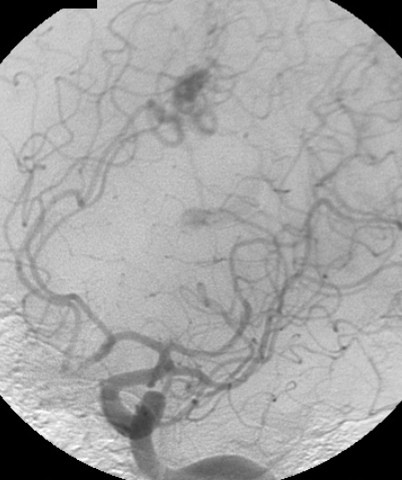
One should keep in mind that in the case of small AVMs, when the initial manifestation is intracerebral hemorrhage, the hematoma might compress and hide the nidus, making the diagnosis through imaging difficult, even for MRI and DSA. Therefore, in such cases, when the first exams are negative for AVMs, they should be repeated after 4-6 weeks, after the reduction of hematoma mass effect.
Intraoperative angiography should be used to evaluate complete extirpation of the lesion. Unexpected residual AVM is shown in 3.7% to 27.3% of intraoperative digital subtraction angiograms after AVM resection cases. [13]
++++
3. Characterization of the diagnosis scores and scales
3.1. Spetzler-Martin Grading System (1986) [95]
The Spetzler-Martin (SM) grading system [95] is a simple and practical scale used worldwide world wide to evaluate surgical risk. It is also very reproducible being useful for comparing different surgical series.
Although it was originally described to assess the risk associated with microsurgical AVM resection, and not for endovascular and radiosurgical risks, it helps in making decisions about all the therapeutic management as will be explained in the treatment.
This grading system is based on three parameters (Table 1) : AVM size, presence of deep venous drainage, and the eloquence of the location. The classification may vary from grade 1 to 5, and the higher the grade, the higher the risk of surgical treatment. A negative point is that there is a great heterogeneity of AVM with the same grade, mainly in the grade III, as discussed later.
3.2. Spetzler-Ponce Classification (2010) [96]
This classification is just a modification of the one described above, gathering AVMs of similar prognosis in three classes :
– Class A : corresponds to grades 1 and 2 of Spetzler-Martin (SM) grading ;
– Class B : corresponds to grades 3 of SM grading ;
– Class C : corresponds to grades 4 and 5 of SM grading.
3.3. Lawton sub-classification of grade III AVM of Spetzler-Martin [45]
Analyzing the grade III AVM, we may observe that inside this group there is a great heterogeneity of AVM anatomy, therapeutic possibilities and risks. The grade III, may be presented in four subtypes : S1V1E1, S2V1E0, S2V0E1, S3V0E0. (S : size, V : venous, E : Eloquent)
Lawton et al. [45] in 2003 described a consecutive series of 174 brain AVMs resected from 174 patients during a period of 4.8 years. The grade III was present in 76 AVMs (45.2%) with an overall risk for new deficit or death of 8%. He proposed a subdivision of the SM III :
– S1V1E1 (III -) : 35 (46.1%) small AVMs. New deficit or death : 2.9%
– S2V1E0 (III) : 14 (18.4%) medium/deep AVMs. New deficit or death : 7.1%
– S2V0E1 (III +) : 27 (35.5%) medium/eloquent AVMs. New deficit or death : 14.8%
– S3V0E0 (III*) : no case. Lawton et al. [45] believe that this subtype is extremely rare or may be a theoretical lesion ; because a nidus exceeding 6 cm in diameter or having a deep draining vein or involve eloquent brain tissue (changing its classification to SM IV).
There was no statistical significant difference between these groups probably because of the relative small number of patients.
3.4. Lawton Supplementary Grading Scale [47]
Lawton et al. [47] proposed a supplement to the SM grading system, and just like it, the supplementary grading system is a tool to assess the risk of AVM resection (Table 2).
Unlike the Spetzler-Martin score, the supplementary score can change with other treatments or with time. Example : after radiosurgery, an AVM can lose its diffuseness, it can rupture during the latency period, and a pediatric patient can transition to an adult patient.
Lawton et al. [47] found that using only the supplementary grading scale it had high predictive accuracy on its own (area under the ROC curve, 0.73 vs. 0.65 for the Spetzler-Martin grading system), and stratified surgical risk more evenly. Therefore, the supplementary grade can be considered separately, or it can be combined with the Spetzler-Martin grade. Patients with supplementary grades ≤3 or combined grades ≤6 stratify into low- or moderate-risk groups that predict acceptably low surgical morbidity.
3.5. Pollock-Flickinger radiosurgery-based grading system for arteriovenous malformations (2002) [84]
Pollock and Flickinger (PF) [84] in 2002 proposed a new grading system for predicting the outcome of patients with AVM submitted to radiosurgery. Because the Spetzler–Martin grade was created to predict microsurgery outcome and did not correlate with excellent patient outcomes (complete AVM obliteration without any new neurological deficit) (p = 0.13) in radiosurgery. [84]
The SM grading system is probably unreliable for radiosurgery and embolization because the complications of these types of treatment differ from those of surgery, and because deeper lesions are not as difficult to access with radiosurgery and embolization, as with microsurgery.
The PF grading system was based on the multivariate analysis of data obtained from 220 patients treated between 1987 and 1991 (Group 1) and tested on a separate set of 136 patients with AVMs treated between 1990 and 1996 (Group 2) at a different center. After this, they proposed the following equation to predict patient outcomes after AVM radiosurgery based on factors relevant to AVM radiosurgery rather than microsurgery :
AVM score = (0.1)(AVM volume in cm3) + (0.02)(patient age in years) + (0.3)(location of lesion)
– AVM volume : π/6 x width x length x height
– Location of lesion : frontal or temporal = 0 ; parietal, occipital, intraventricular, corpus callosum, cerebellar = 1 ; or basal ganglia, thalamic, or brainstem = 2.
The score interpretation was :
– Score of 1 or less : > 95% patients had an excellent outcome ;
– Score of 1.25 : > 80% patients had an excellent outcome ;
– Score of 1.5 : > 70% patients had an excellent outcome ;
– Score of 1.75 : > 60% patients had an excellent outcome ;
– Score of 2 : > 50% patients had an excellent outcome ;
– Score greater than 2 : < 40% patients had an excellent outcome.
Excellent patient outcome was defined as complete AVM obliteration without any new neurological deficit. One hundred twenty-one (55%) of 220 Group 1 patients had excellent outcomes. In addition, seventy-nine (58%) of 136 Group 2 patients had excellent outcomes.
The authors concluded that the system allows a more accurate prediction of outcomes from radiosurgery to guide choices between surgical and radiosurgical management for individual patients with AVMs.
Although, there are some important limitations highlighted by the authors :
– This system was created to predict patient outcomes after a single radiosurgery procedure.
– Complications after AVM radiosurgery may occur many years after angiographically verified obliteration. [41, 111] Hence, the final results of AVM radiosurgery may change with a longer follow up.
– The grading system was developed and tested at centers at which only the gamma knife is used to perform radiosurgery
3.6. Pollock-Flickinger modified radiosurgery-based grading system for arteriovenous malformations (2008) [85]
In 2008, Pollock-Flickinger proposed a simplification of their original grading system, using location as a two-tiered variable. They analyzed 220 patients who underwent AVM radiosurgery between 1987 and 1992 and 247 patients who underwent AVM radiosurgery between 1990 and 2001 at another center.
They proposed the following equation :
– AVM score = (0.1)(AVM volume in cm3) + (0.02)(patient age in years) + (0.5)(location of lesion)
– Location of lesion : frontal, temporal, parietal, occipital, intraventricular, corpus callosum, cerebellar = 0 (other location) ; basal ganglia, thalamus, brainstem = 1 (deep location).
The score interpretation was :
– Score ≤ 1 : 89% patients had an excellent outcome and 0% had a decline in Modified Rankin Scale ;
– Score of 1.01-1.50 : 70% patients had an excellent outcome and 13% had a decline in Modified Rankin Scale ;
– Score of 1.5-1.99 : 64% patients had an excellent outcome and 20% had a decline in Modified Rankin Scale ;
– Score ≥ 2 : 46% patients had an excellent outcome and 36% had a decline in Modified Rankin Scale ;
Excellent patient outcome was defined as complete AVM obliteration without any new neurological deficit.
They found no difference between the original and modified scale with regard to AVM obliteration without new neurological deficits (p = 0.53) or decline in Modified Rankin Scale (p = 0.56).
As the first PF grade, this new grade was developed at centers performing gamma knife radiosurgery, but it has also been demonstrated to work equally well with linear accelerator radiosurgery.[4, 49, 85, 114]
++++
VII. Treatment
Treatment aimed at preventing hemorrhage, controlling seizures and reversing any progressive neurological deficit, in order to increase survival with the least morbidity possible. In most cases, this is possible by means of complete excision of the lesion. Although this may not always be the best option for the patient depending : on his/her overall condition, on the AVM Spetzler-Martin grade, and on the experience of the treatment team (neurosurgeon, neuroradiologist or radiosurgeon). Thus the risks of conservative versus invasive treatments should be considered.
There are no randomized studies to guide such a decision, which means each case must be carefully analyzed by a multidisciplinary and experienced team. Partial treatment of AVMs has shown proven not to be not beneficial, and may result in an increase in the risk of hemorrhage, according to some studies. So when planning to treat an AVM the total resection should always be considered as the only option. Although there are some exceptions, and sometimes the partial treatment is very important :
– Cases of giant AVMs with risk factors such as intranidal aneurysms that can be treated with the occlusion of the aneurysms.
– Progressive neurological deficit secondary to high flow fistulas with steal flow symptoms that can be treated with the occlusion of the fistulas.
– Intractable headache that can be treated with occlusion of the meningeal arteries.
++++
1. Ruptured AVM versus unruptured AVM
In patients with incidental AVMs, the benefits of treatment, the best therapeutic modality and the overall long-term outcome following treatment are controversial. The patient’s clinical situation and the natural history of the lesion are perhaps the most important considerations when deciding whether and how to treat [12]. The objective is to treat only the patient with AVM in which the perceived risks of treatment are less than the presumed natural history risk.
As reported in the natural history the previous history of bleeding is fundamental in therapeutic decision, because it increases the risk of rebleeding.
There are some extreme situations in which the decision to treat (young patient with history of bleeding and small or medium AVM) or not to treat (patient with more than 50 years, without history of bleeding and large AVM) are very clear. However, the majority of cases are not so easy and classifications may help us in treatment decisions.
Following the analysis of malformations according to the Spetzler-Martin grading scale and Lawton sub-classification of grade III AVM of Spetzler-Martin, we follow the guidelines at Figure 5 and Figure 6 as screening for treatment. Therefore the AVM treatment must be individualized and many other factors must be taken into account such as : patient’s wishes, age of the patient, AVM’s risk factors for rupture, experience of the team.

1 Spetzler-Martin Grading System (1986) [95]
2 Lawton sub classification of grade III AVM of Spetzler-Martin [45]
3 Usually surgery. Radiosurgery for patients with poor clinical condition. Embolization for AVMs with a single feeding artery (Item VII-2.1.).
4 Usually Surgery. Surgery when S2V0E0. Surgery or radiosurgery when S1V1E0. Radiosurgery when S1V0E1. (S=size ; V=Drainage Venous ; E= Eloquent). Embolization for small AVMs with a single feeding artery. (Item VII-2.1.).
5 Item VII-2.2.1.
6 Item VII-2.2.2.
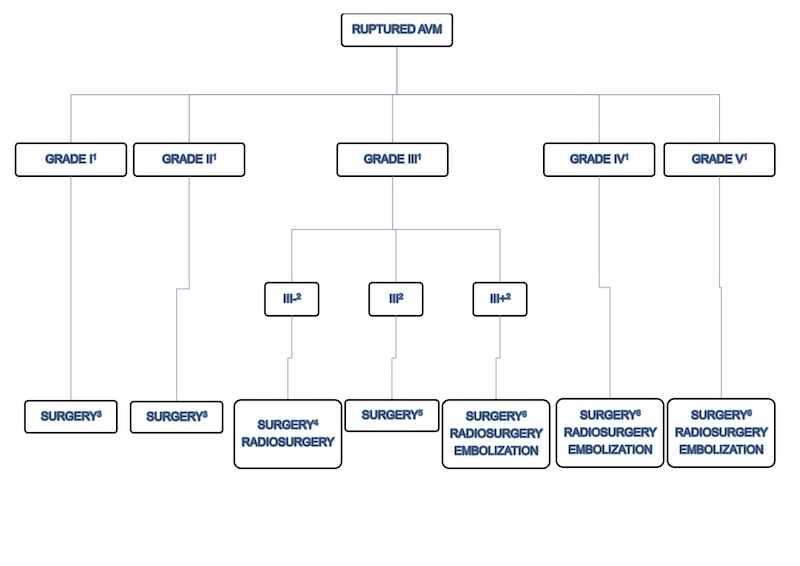
1 Spetzler-Martin Grading System (1986) [95]
2 Lawton sub classification of grade III AVM of Spetzler-Martin [45]
3 Item VII-2.1.
4 Item VII-2.2.1.
5 Item VII-2.2.2.
6 Usually conservative treatment. Partial treatment in patients of elevated risk factors such as intra-nidal aneurysm and high flow arteriovenous fistulas with progressive neurological deficit. (Item VII 2.2.3. and Item 2.3.)
++++
2. Classification and treatment
The Spetzler-Martin (SM) and Spetzler-Ponce (SP) grading systems [95, 96], as previously described, can be a guide for the therapeutic decision :
2.1. SM Grades 1 (Figure 7) and 2 (Figure 8) or SP Class A :
The treatment of choice is microsurgical resection, but embolization or radiosurgery may be considered as well. In the literature, the risk of morbidity (deficit risk) in the postoperative period ranges from 0.7 to 5% for microsurgery. [25, 32, 92, 95] Whereas preoperative embolization in class A is associated with a fall in the modified ranking Rankin scale (mRS) of up to 16% [106]. Radiosurgery has been related, in some studies, to an increase in the bleeding rate of low grade when compared to high grade [40].
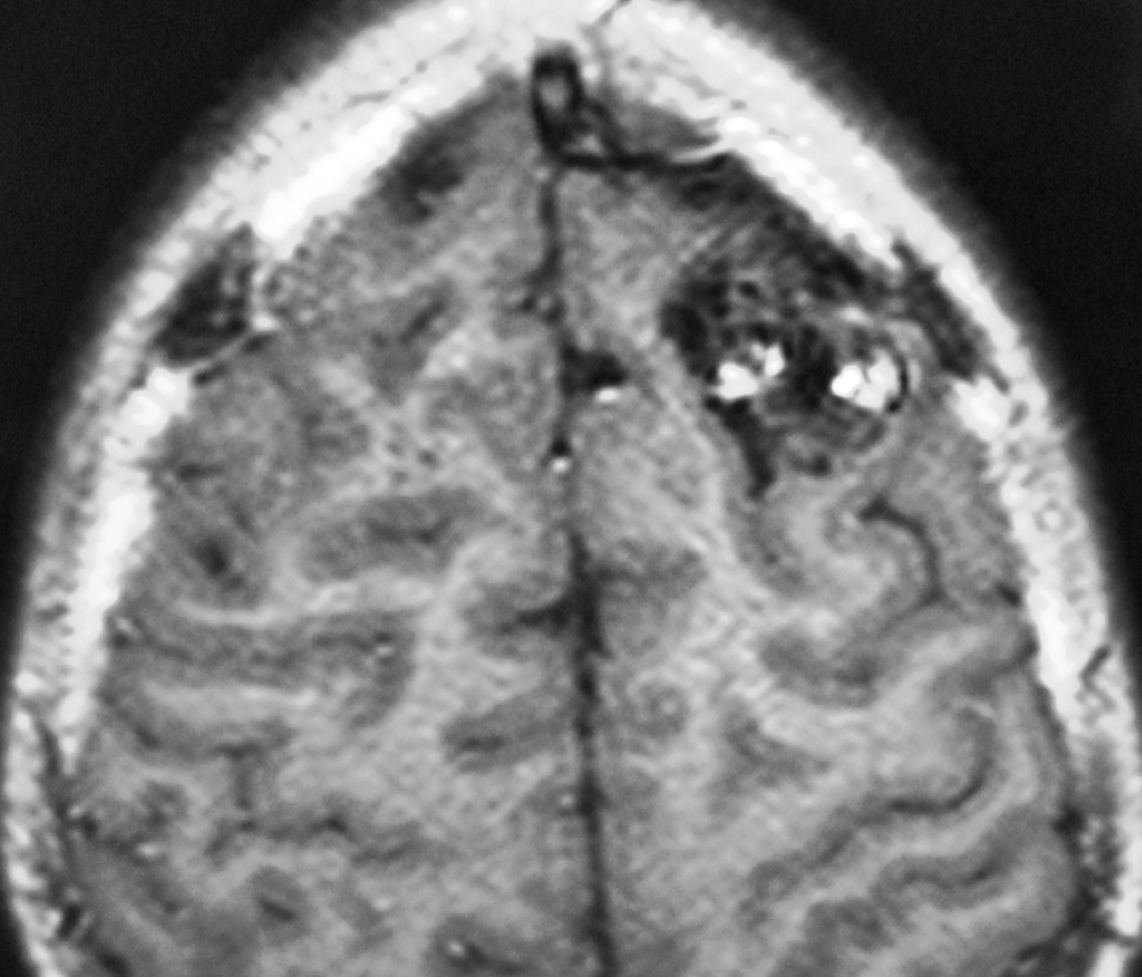
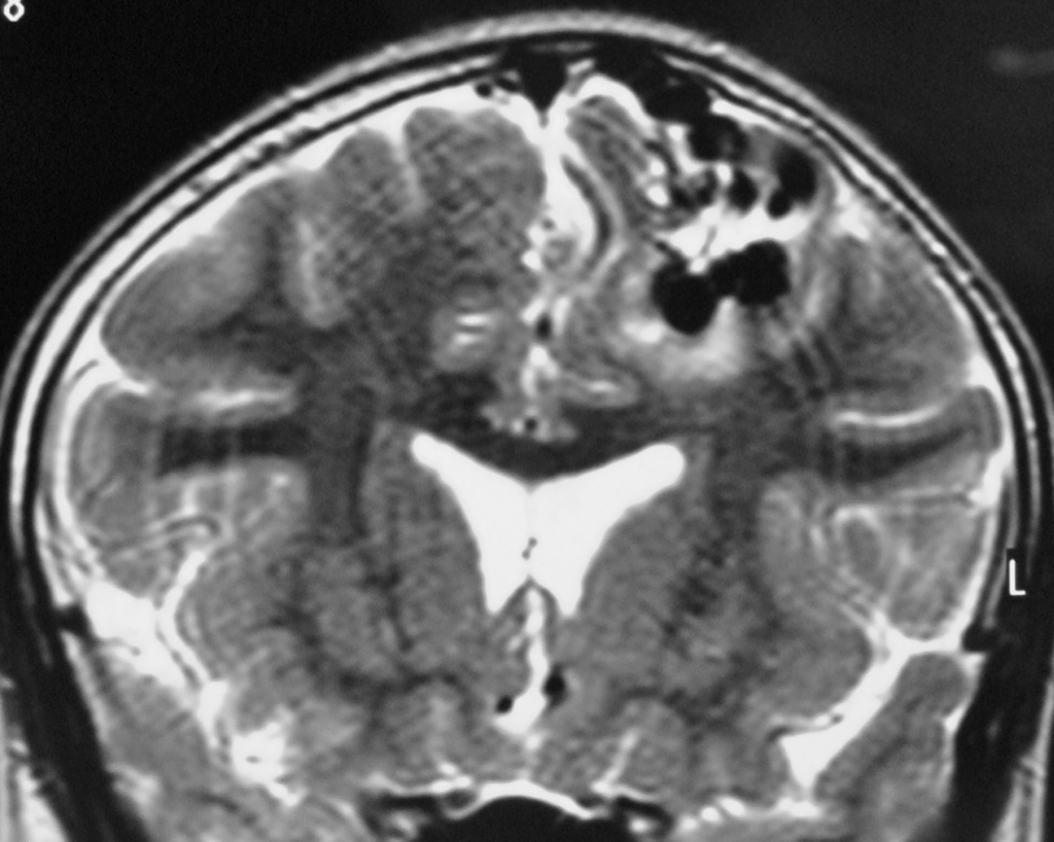
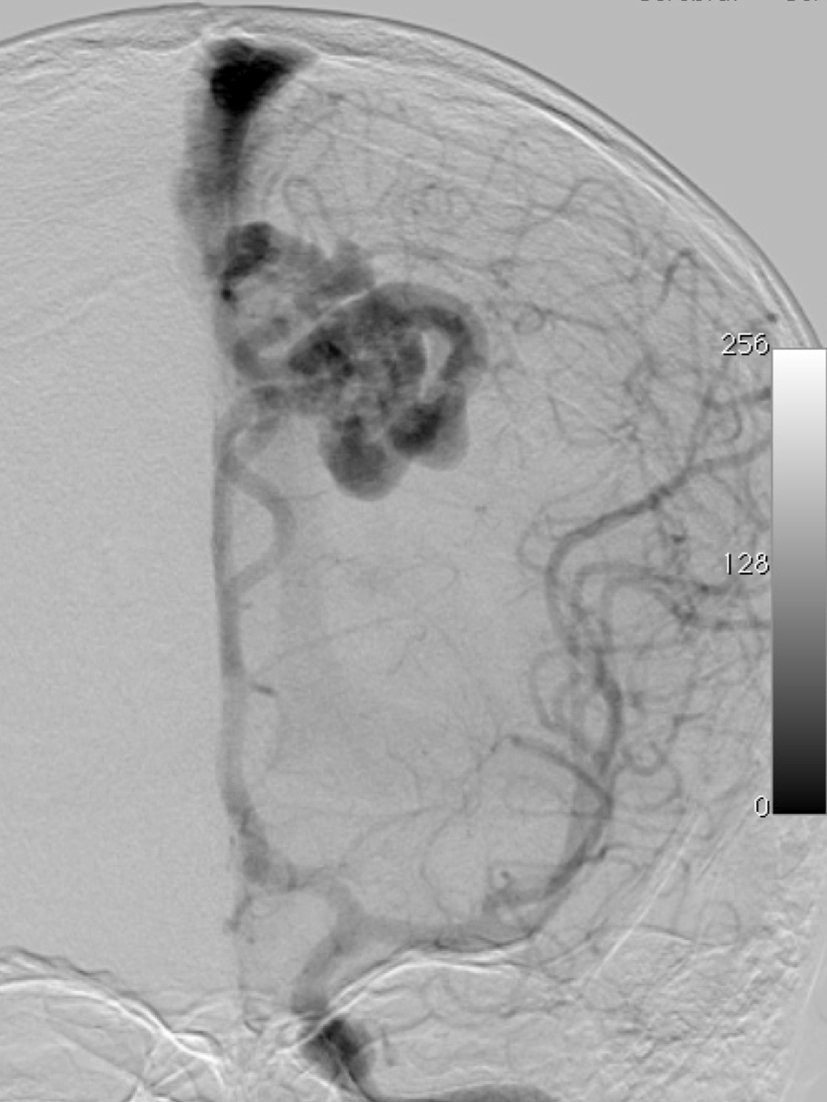
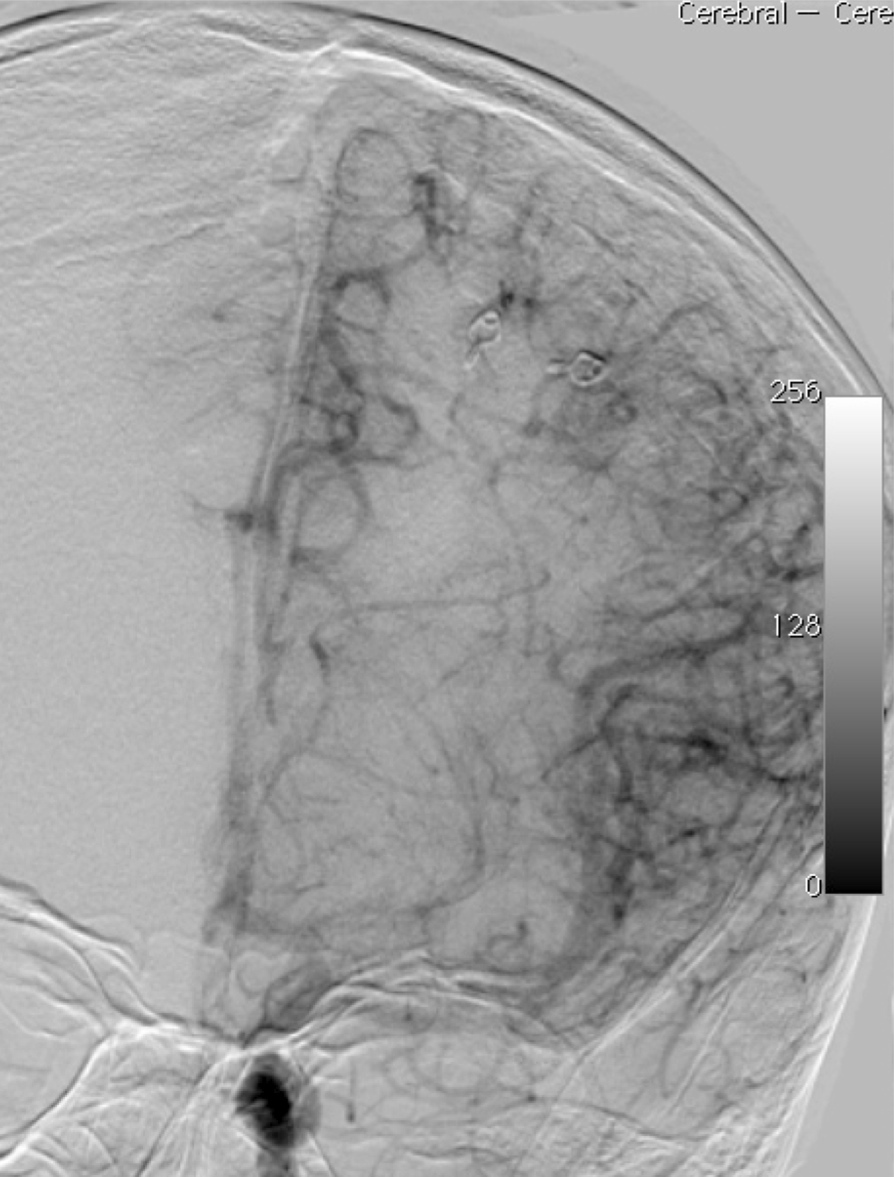
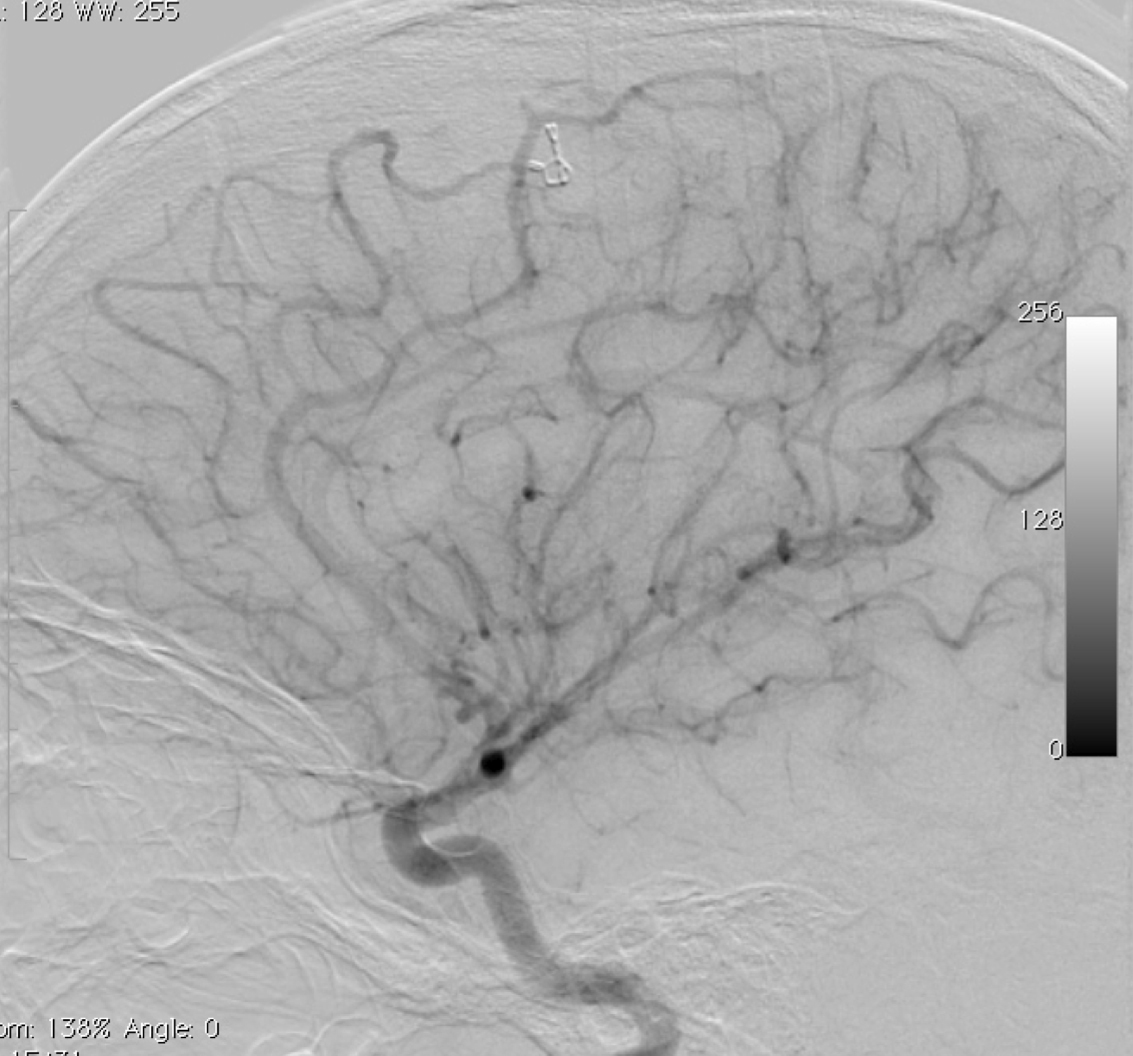

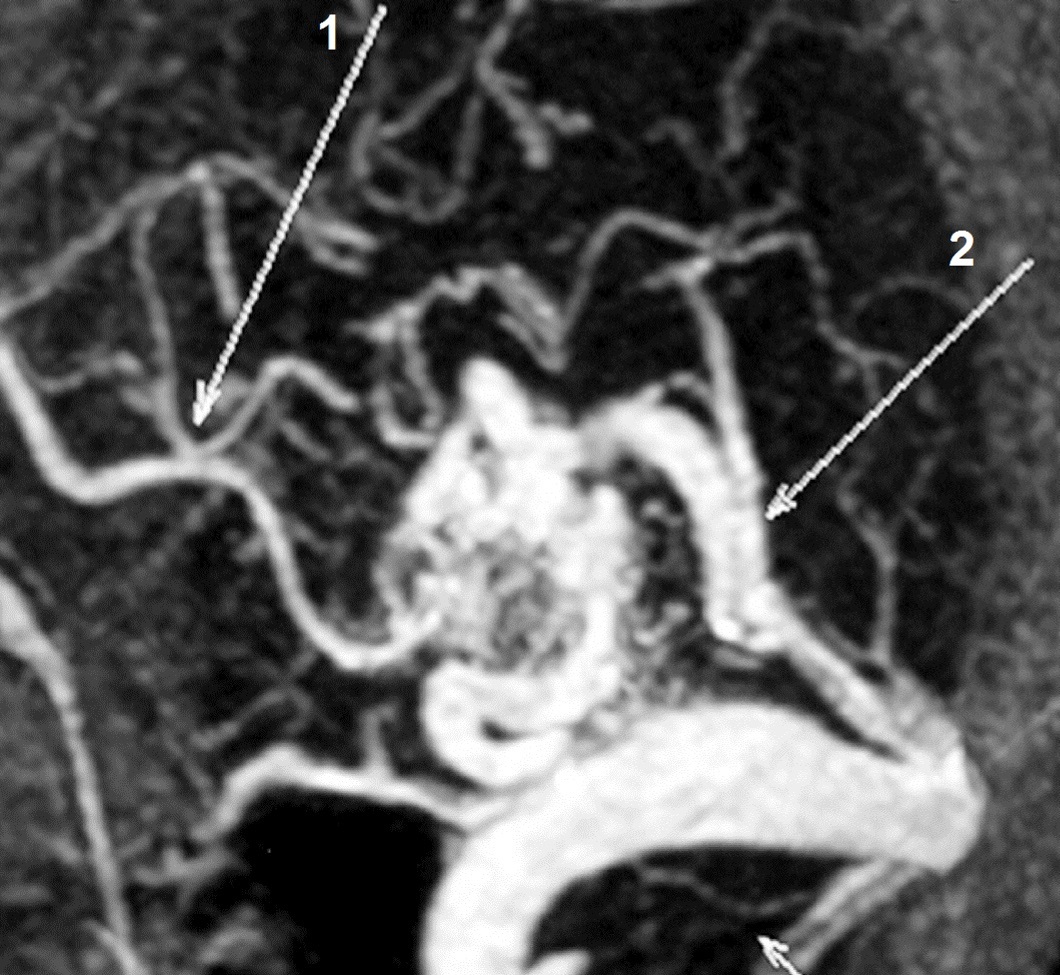
b) Computed tomography angiography (CT angiography) showing an AVM fed by a branch of the left posterior cerebral artery (PCA) (arrow 1) and draining to the sigmoid sinus.
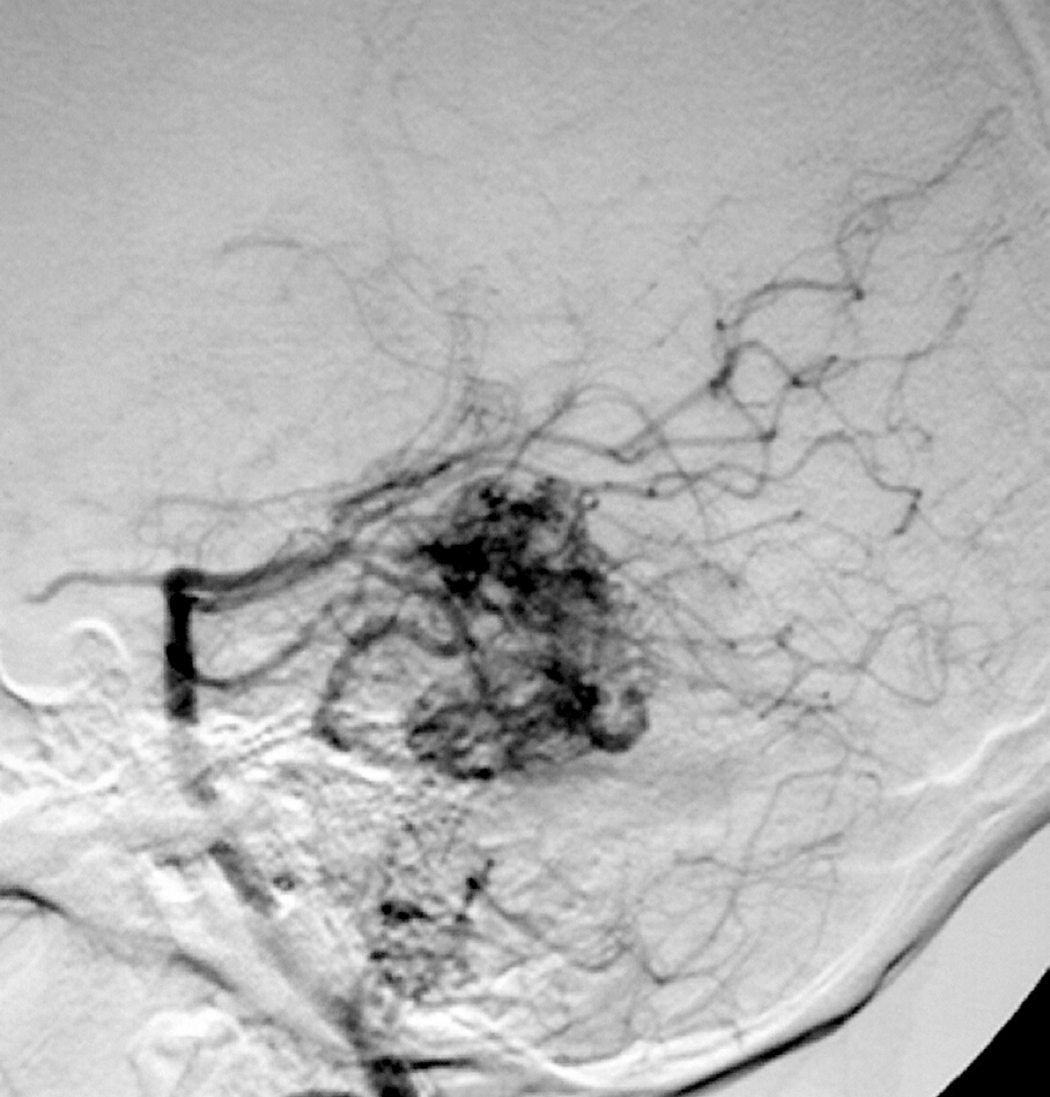
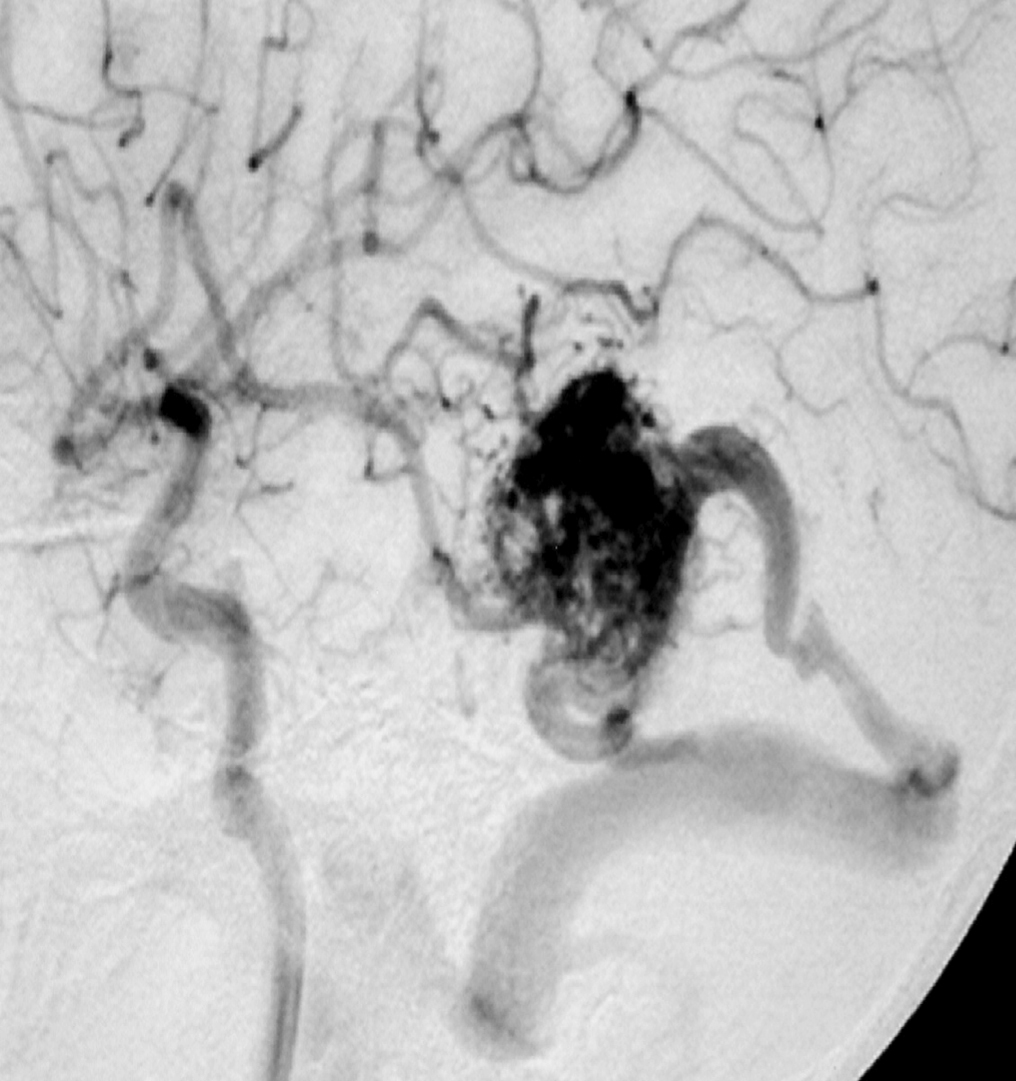
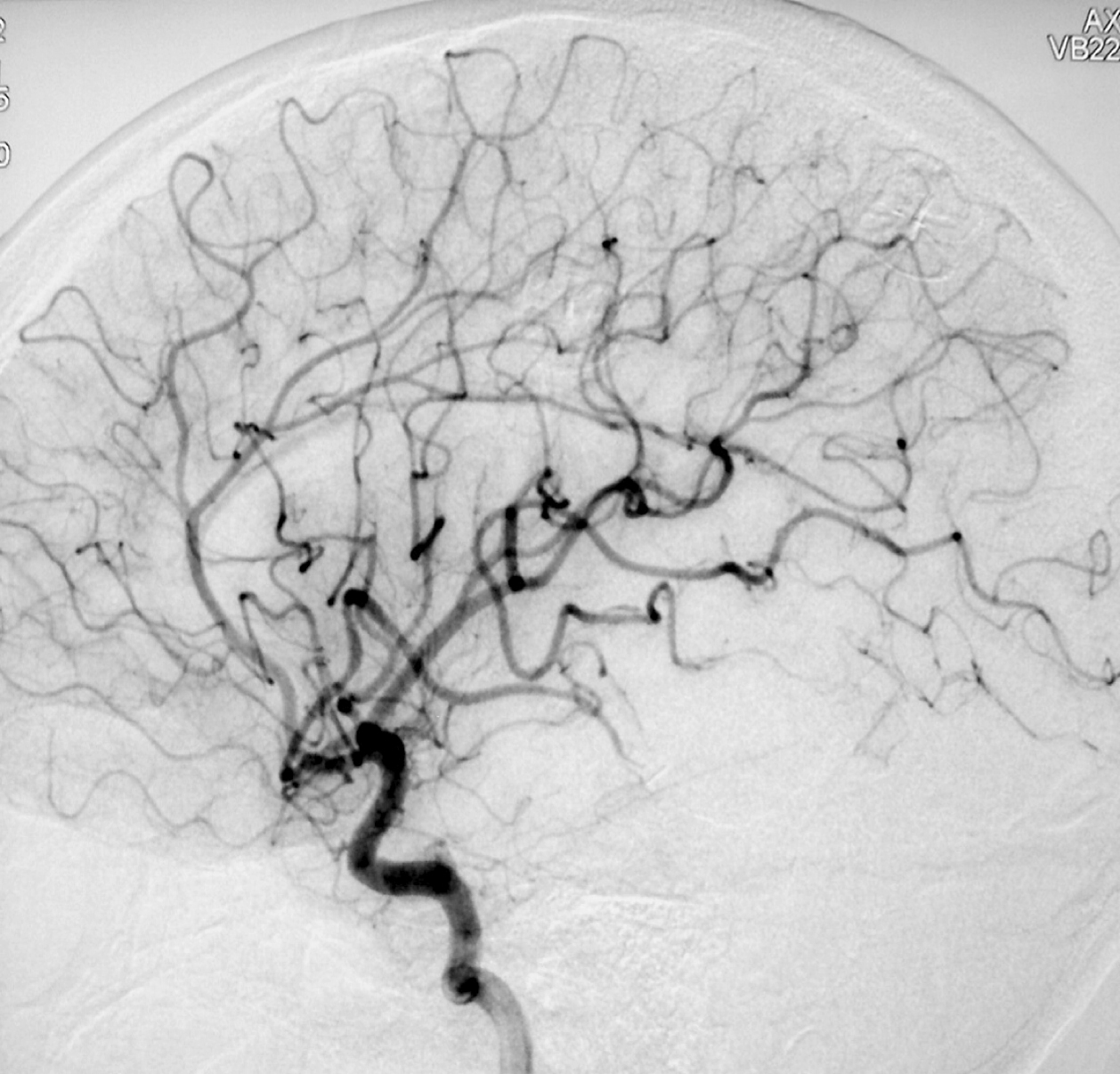
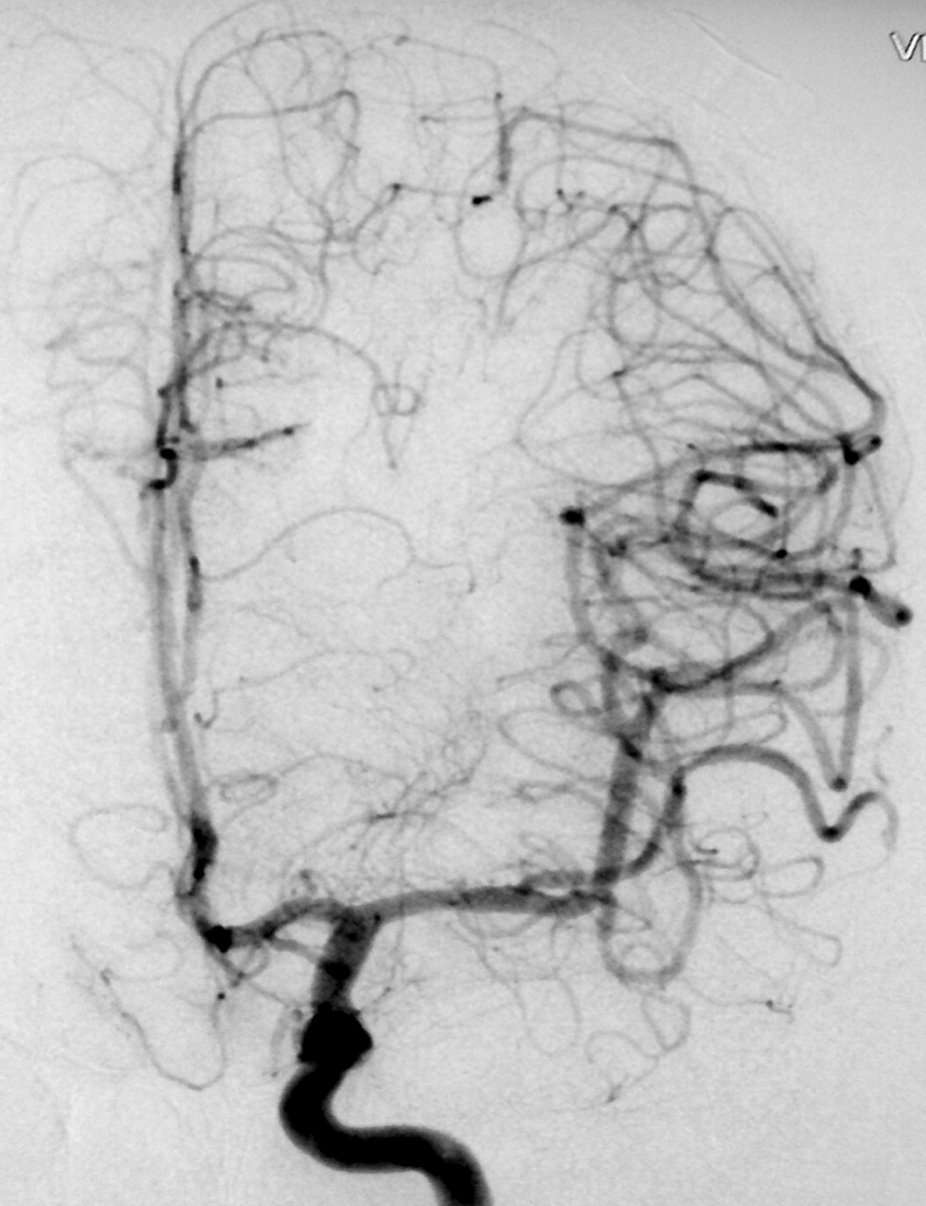
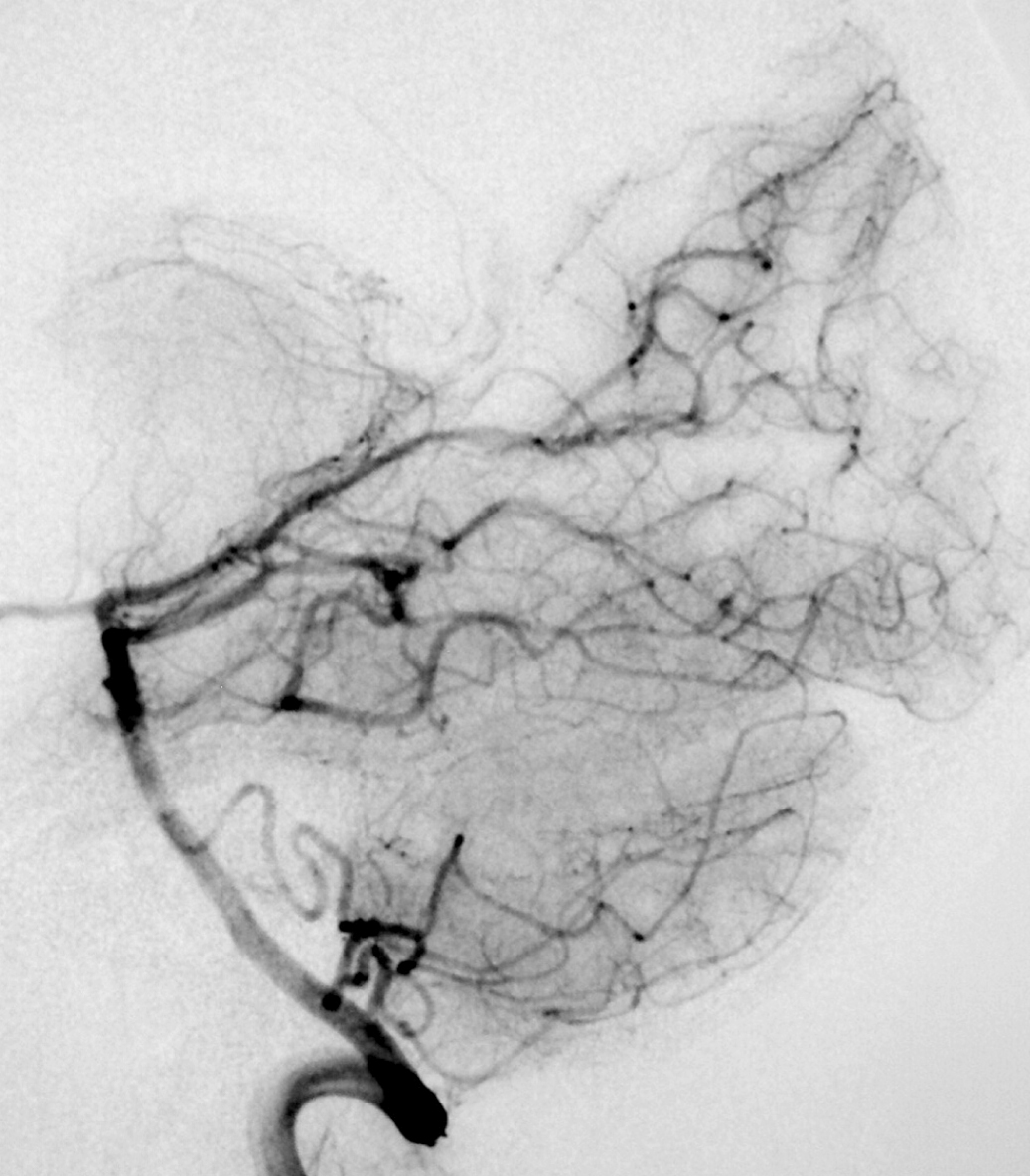
++++
2.2. SM Grade 3 (Figure 9) or SP Class B :
Depending on the size and location, it can be treated with surgery, radiosurgery, embolization, or a combination of these methods (multimodality treatment). The postoperative morbidity (deficit risk) varies from 8 to 16%. [32, 45, 95]
Using the Lawton sub-classification. [45] :
2.2.1. S1V1E1 (III -) : surgical risk similar to that of low-grade AVMs (SP A) (new deficit or death : 2.9%) - can be safely treated with microsurgical resection. These lesions may also be treated with radiosurgery. Although, we agree with Lawton et al : when there is a surgical corridor (like the sylvian fissure, interhemispheric fissure, basal cistern, etc) we recommend microsurgical resection. Microsurgical and radiosurgical morbidity rates are often comparable but microsurgery has immediacy and an efficacy of nearly 100%.
Radiosurgery also has high rates of obliteration in small AVM (from 81 to 90%). The main disadvantage of radiosurgery is that complete obliteration takes from 1 to 3 years after the procedure.
2.2.2. S2V1E0 (III) : intermediate surgical risks (new deficit or death : 7.1%) - carefully individualized treatment.
2.2.3. S2V0E1 (III +) : surgical risk similar to that of high-grade AVMs (SP C) (new deficit or death : 14.8%) - best managed conservatively
2.3.4. S3V0E0 (III*) : extremely rare or does not exist
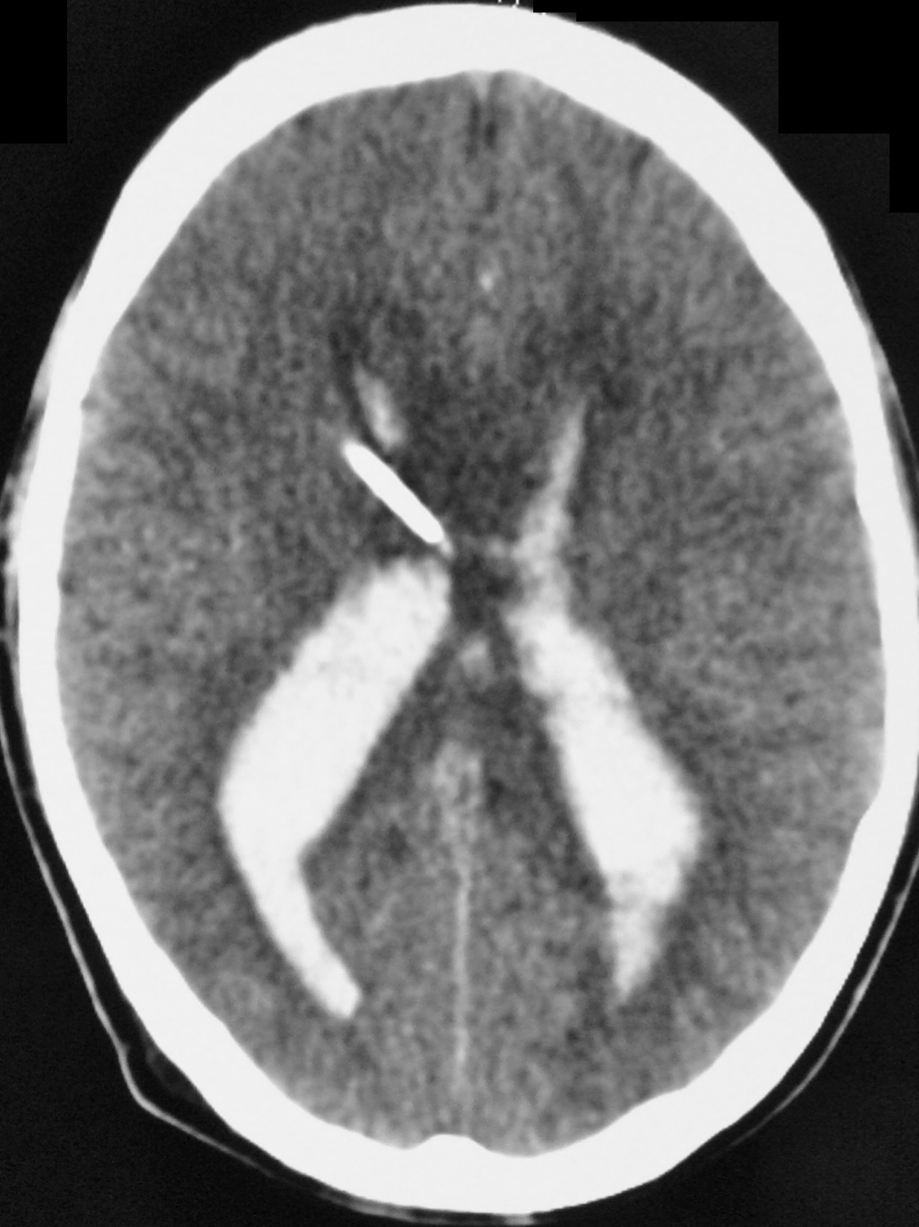
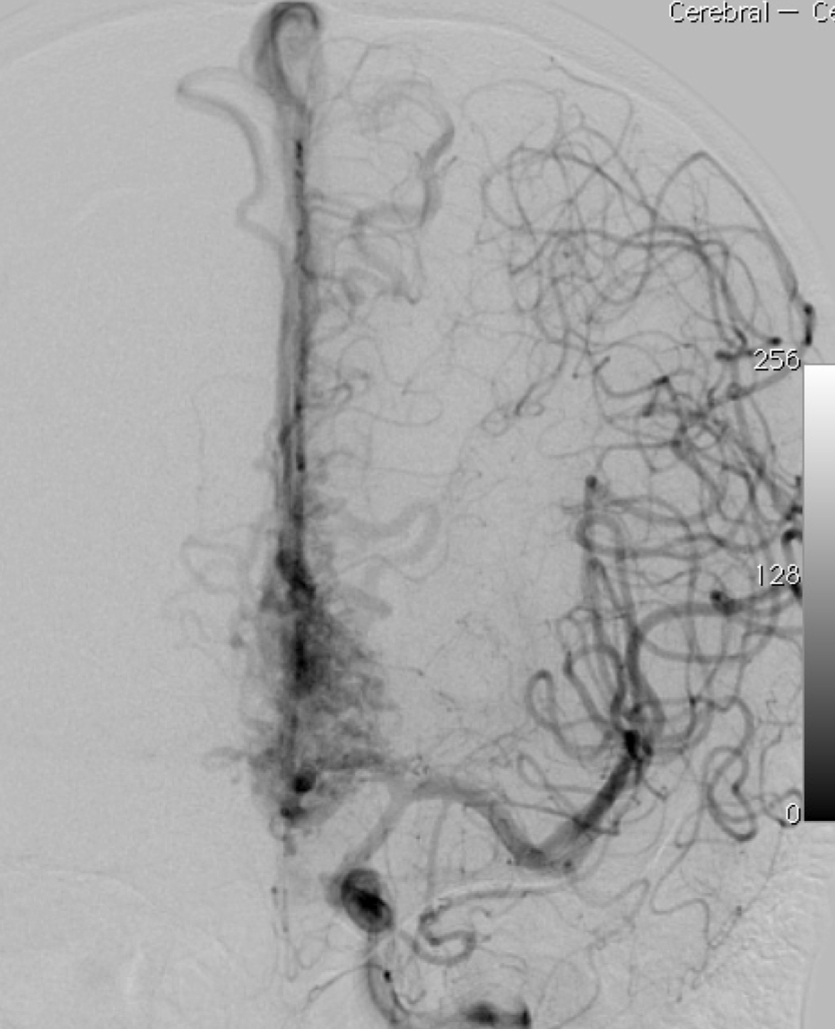

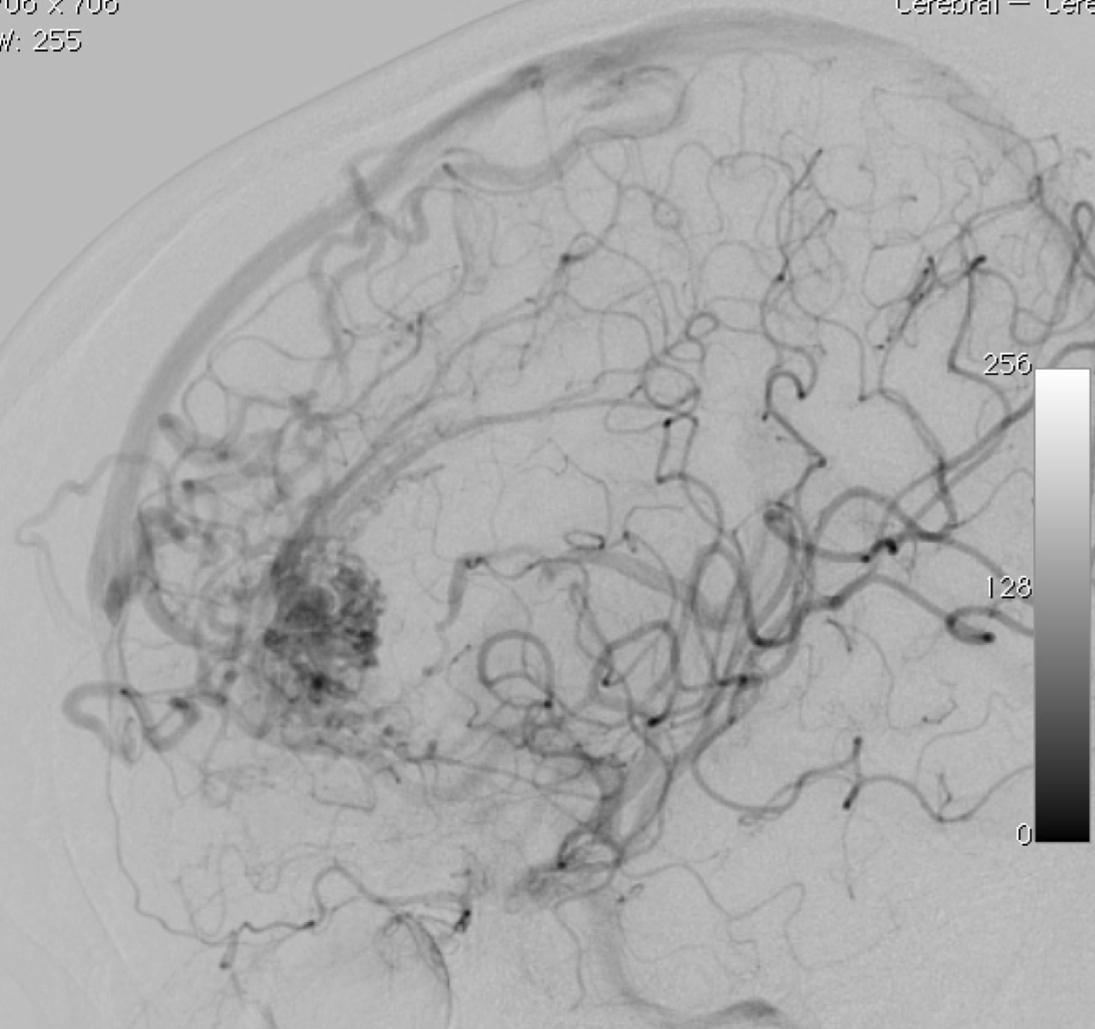
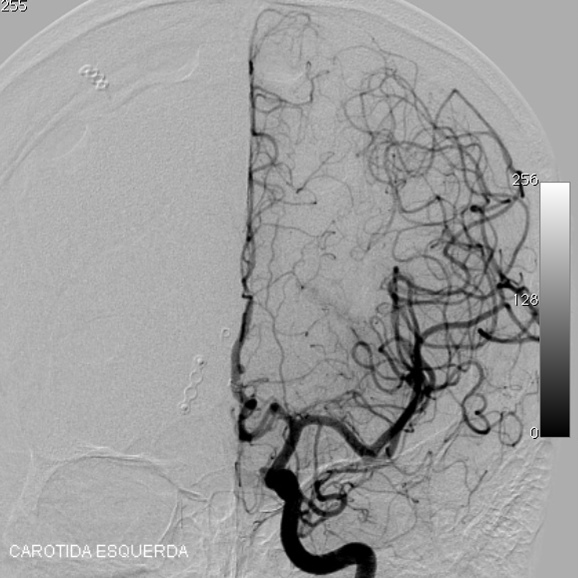
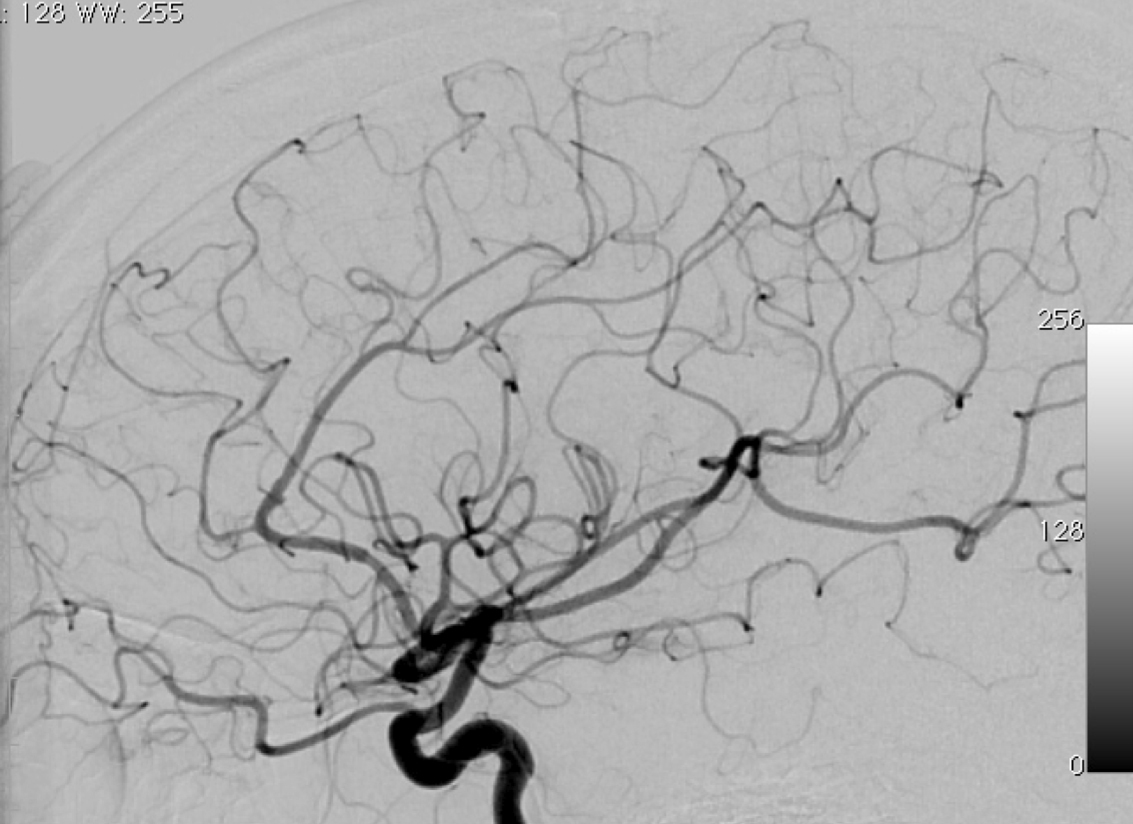
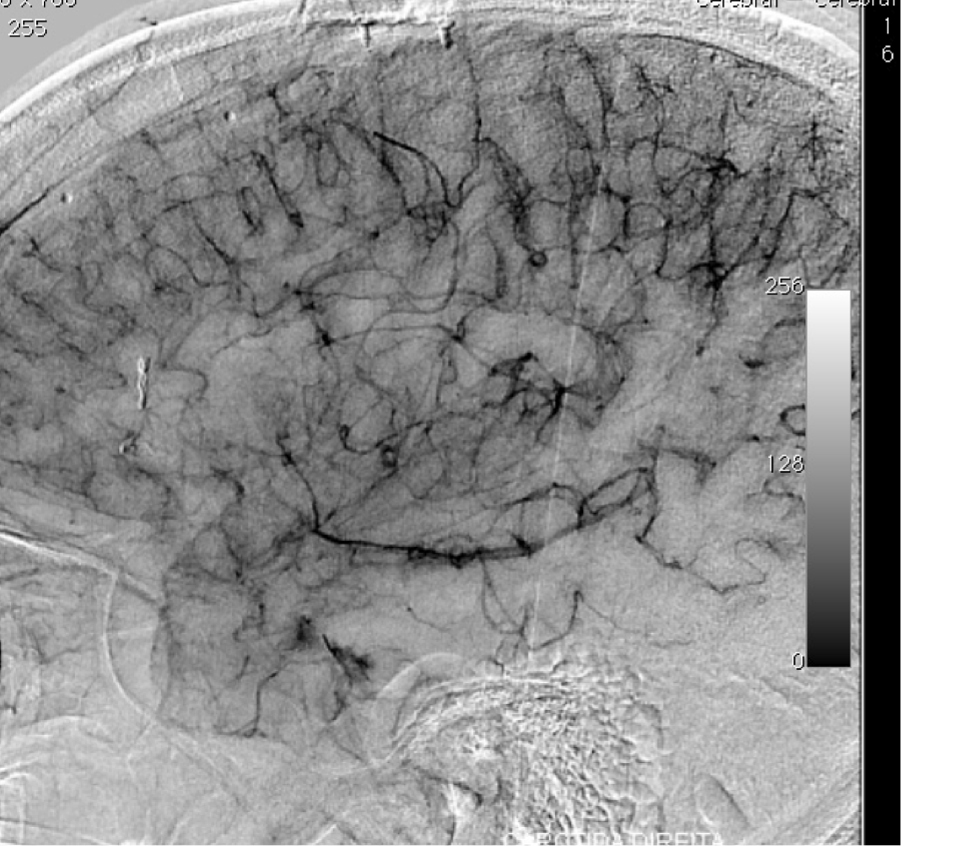
++++
2.3. SM Grades 4 (Figure 10) and 5 (Figure 11) or SP Class C :
Usually conservative treatment. Multimodality treatment is proposed in specific cases such as very young patients, with recurrent hemorrhages or progressive neurological deficit. Partial treatment is also possible in cases of elevated risk factors such as intra-nidal aneurysm and high flow arteriovenous fistulas with progressive neurological deficit. High post-treatment morbidity (deficit risk) rate : 12 to 38%. [25, 32, 95]
The Lawton et al. [47] supplementary grading scale may help in treatment decision. As they proposed, an AVM with a low Spetzler-Martin grade (grade I – III) may be favorable for microsurgical resection, and a low supplementary grade (I – III) may strengthen the recommendation for surgery. Conversely, an AVM with a high Spetzler-Martin grade (IV – V) may be unfavorable for microsurgical resection, and a high supplementary grade (IV – V) may strengthen the recommendation for non-operative management. In cases of matched Spetzler-Martin and supplementary grades, the supplementary grading system has a confirmatory role and may not alter management decisions. However, in cases of mismatched Spetzler-Martin and supplementary grades, the supplementary grading system may alter management decisions and therefore has a more important role.
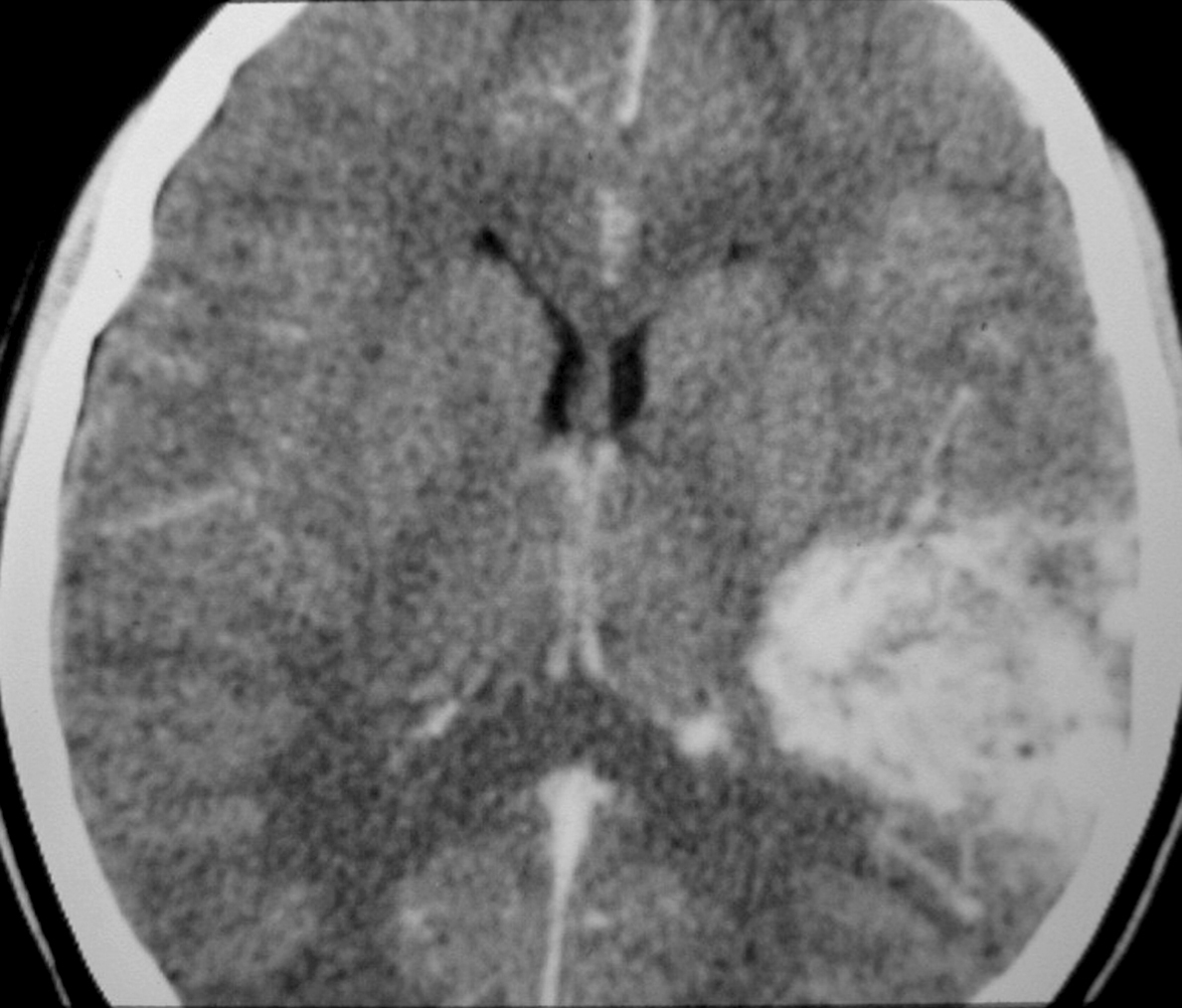
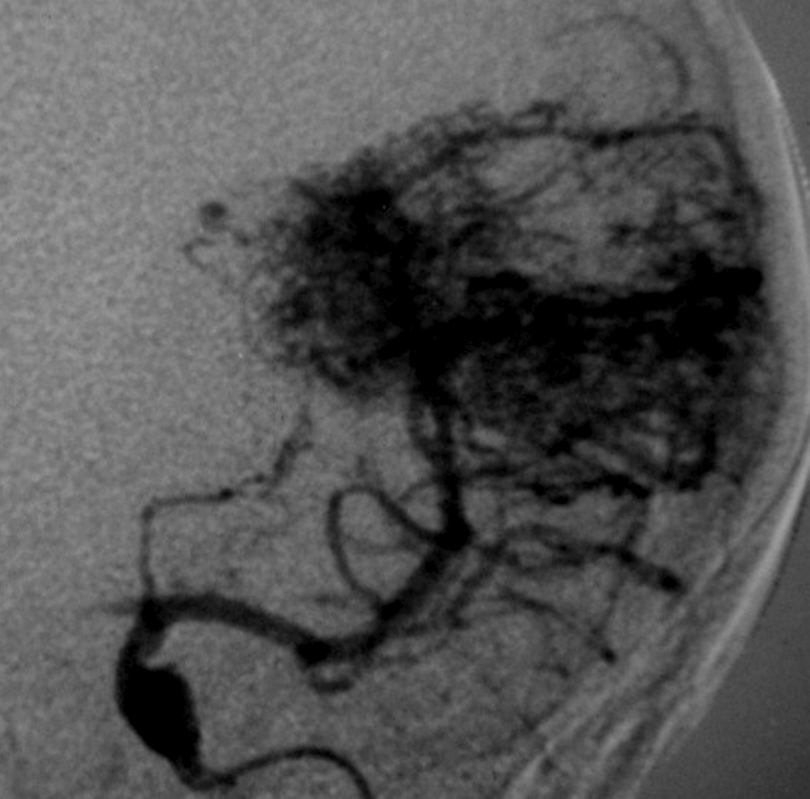

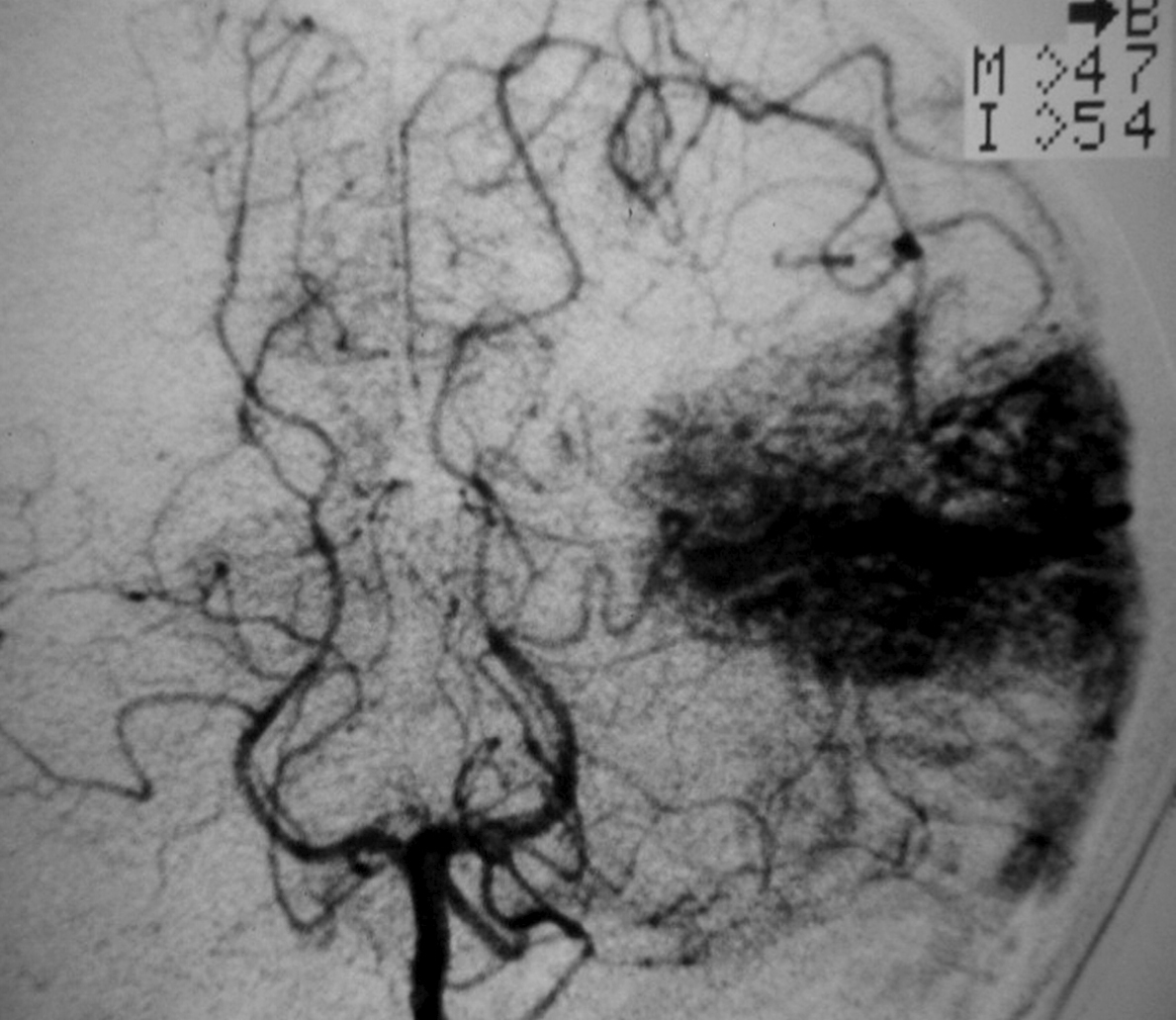
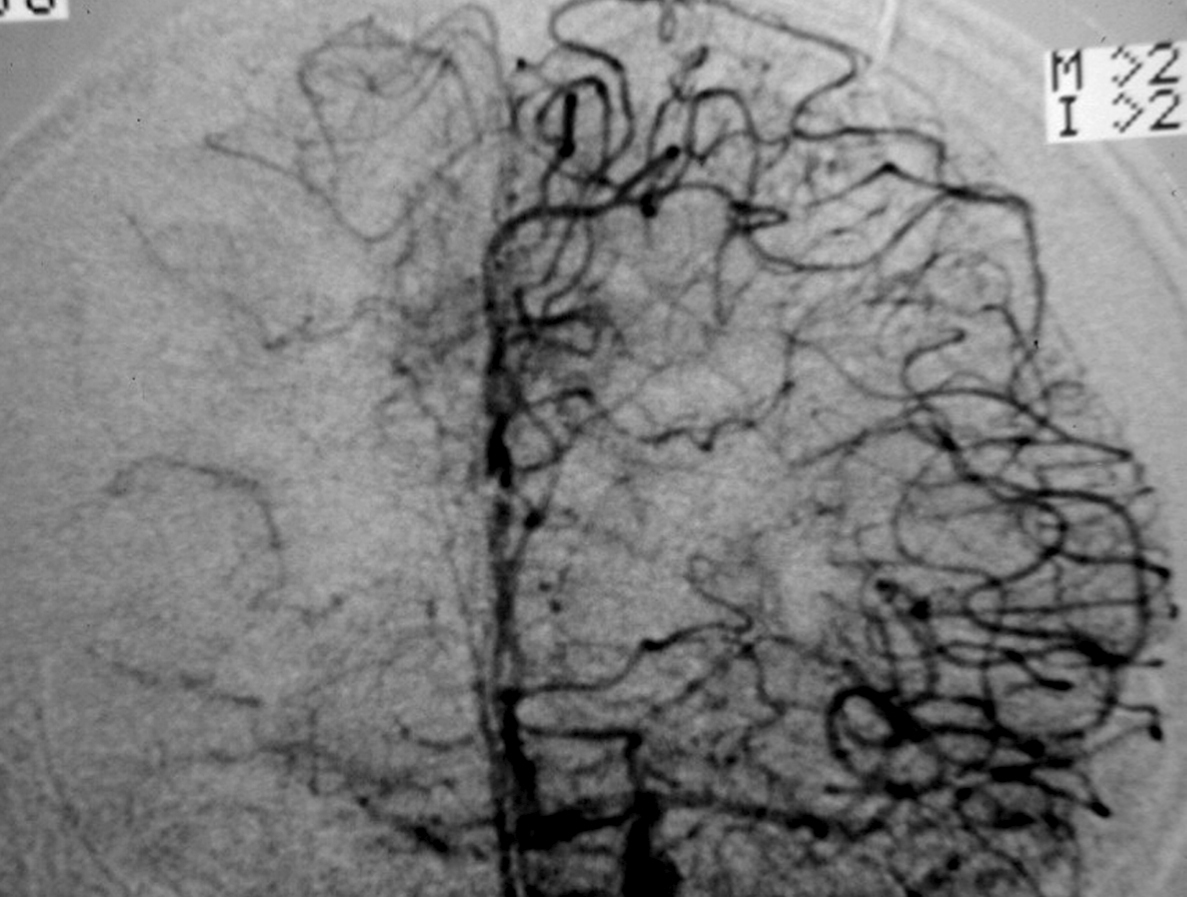
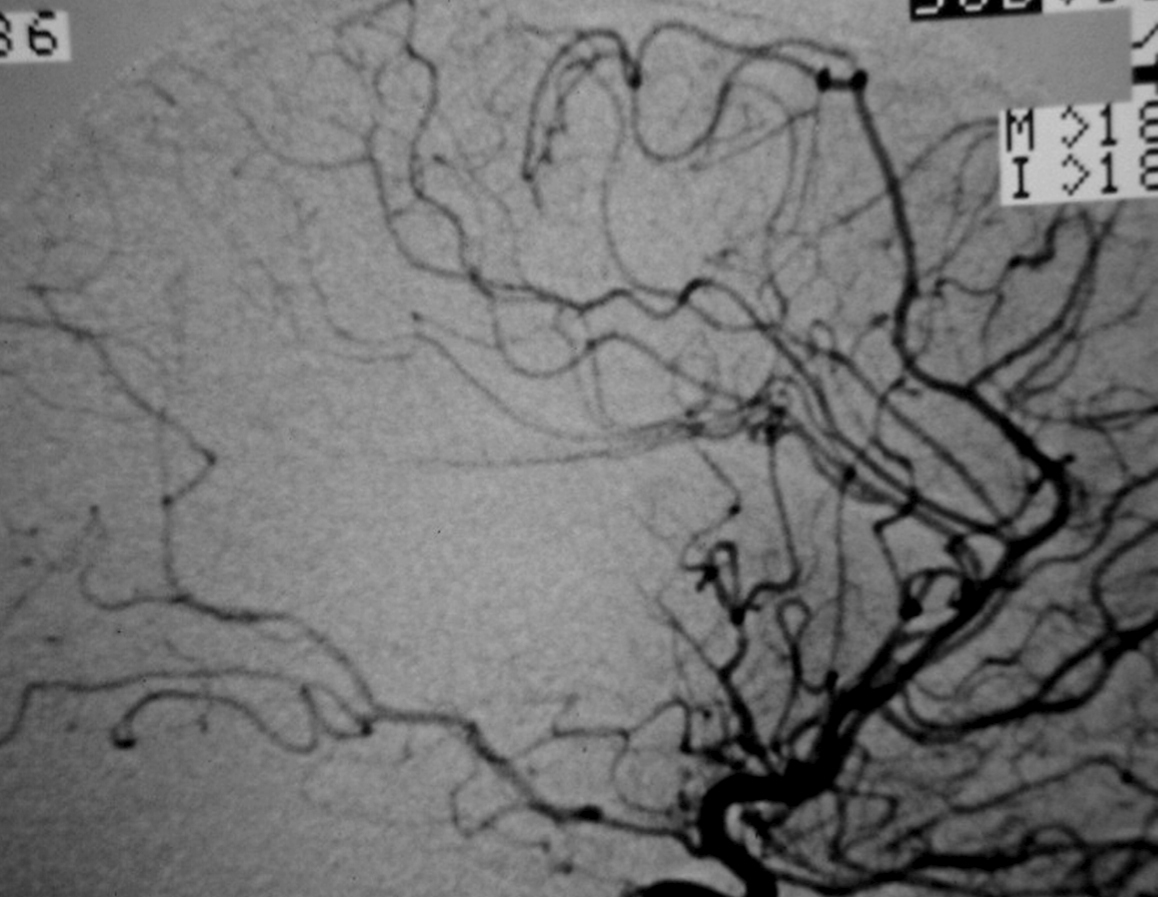
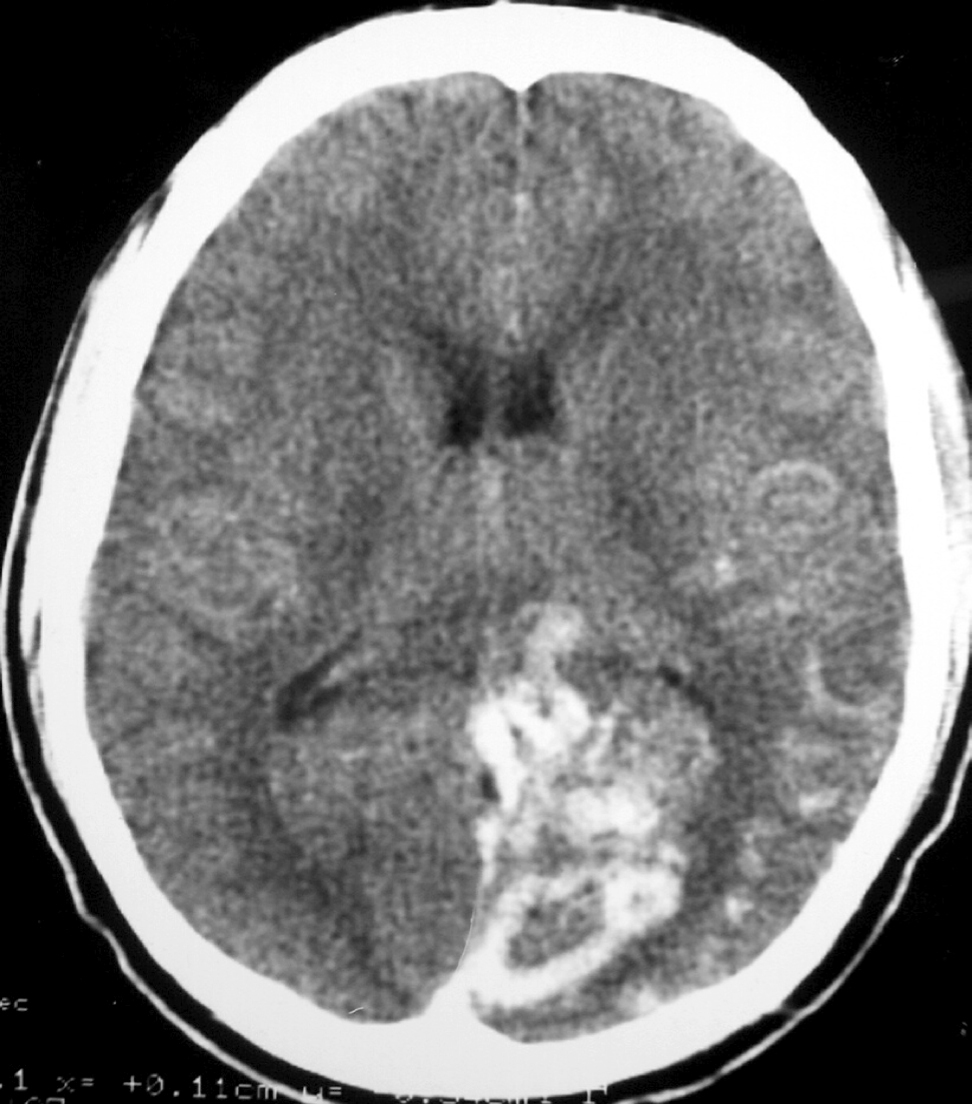
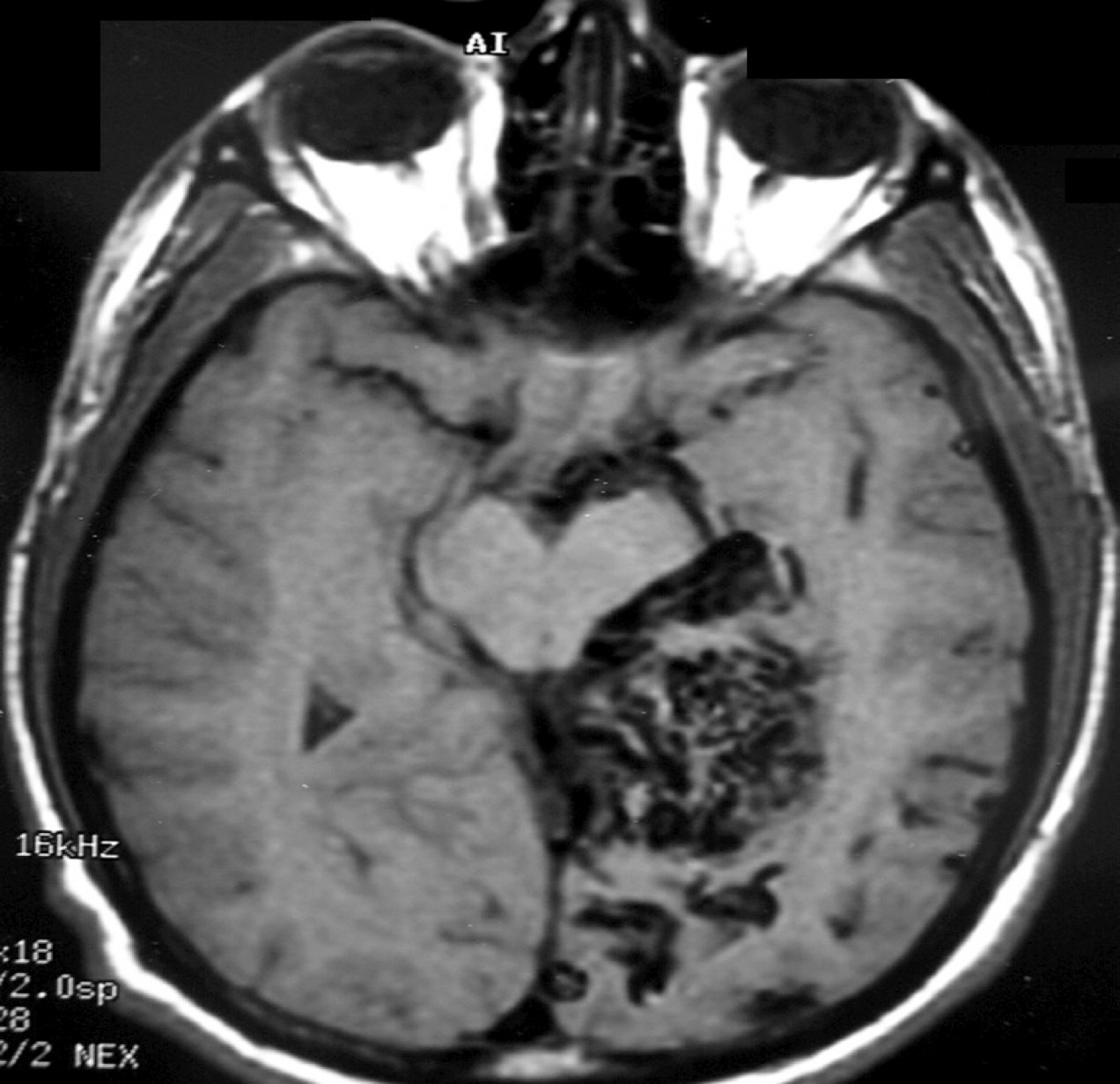
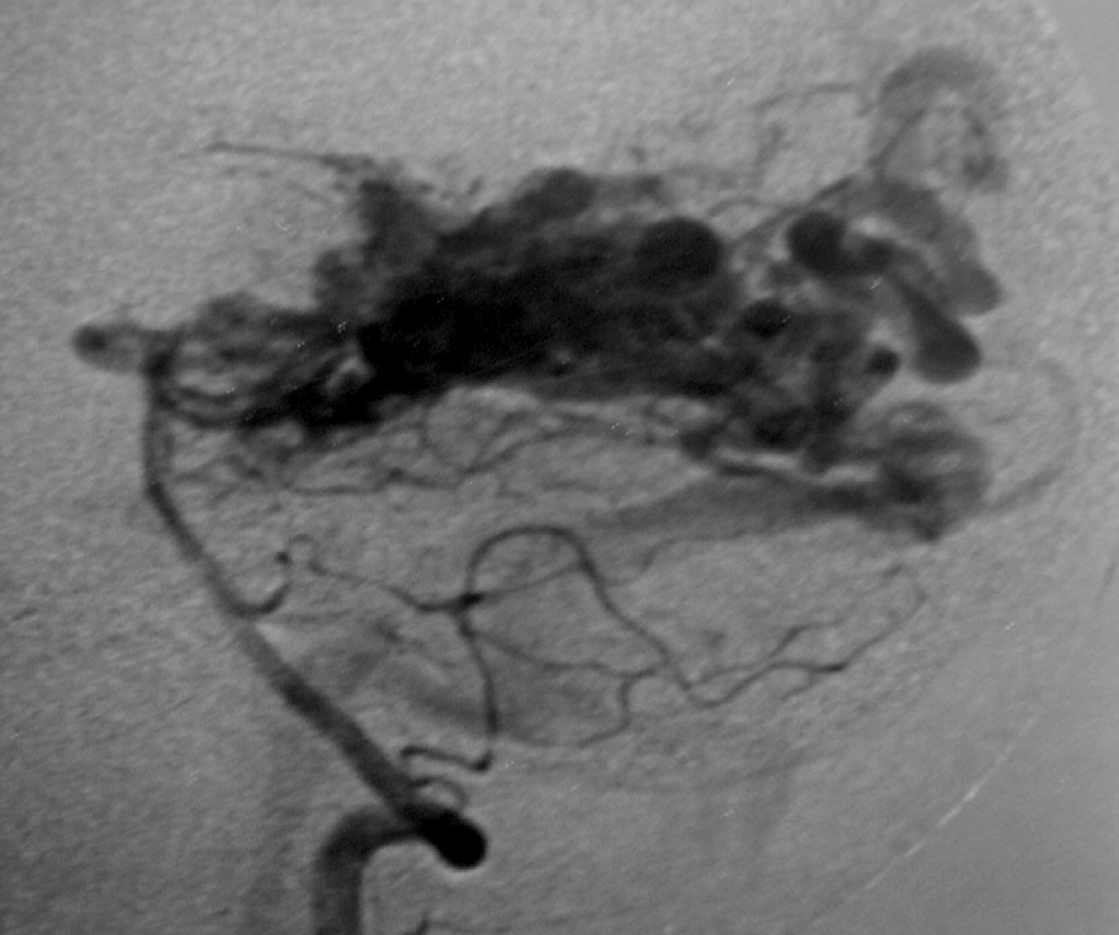
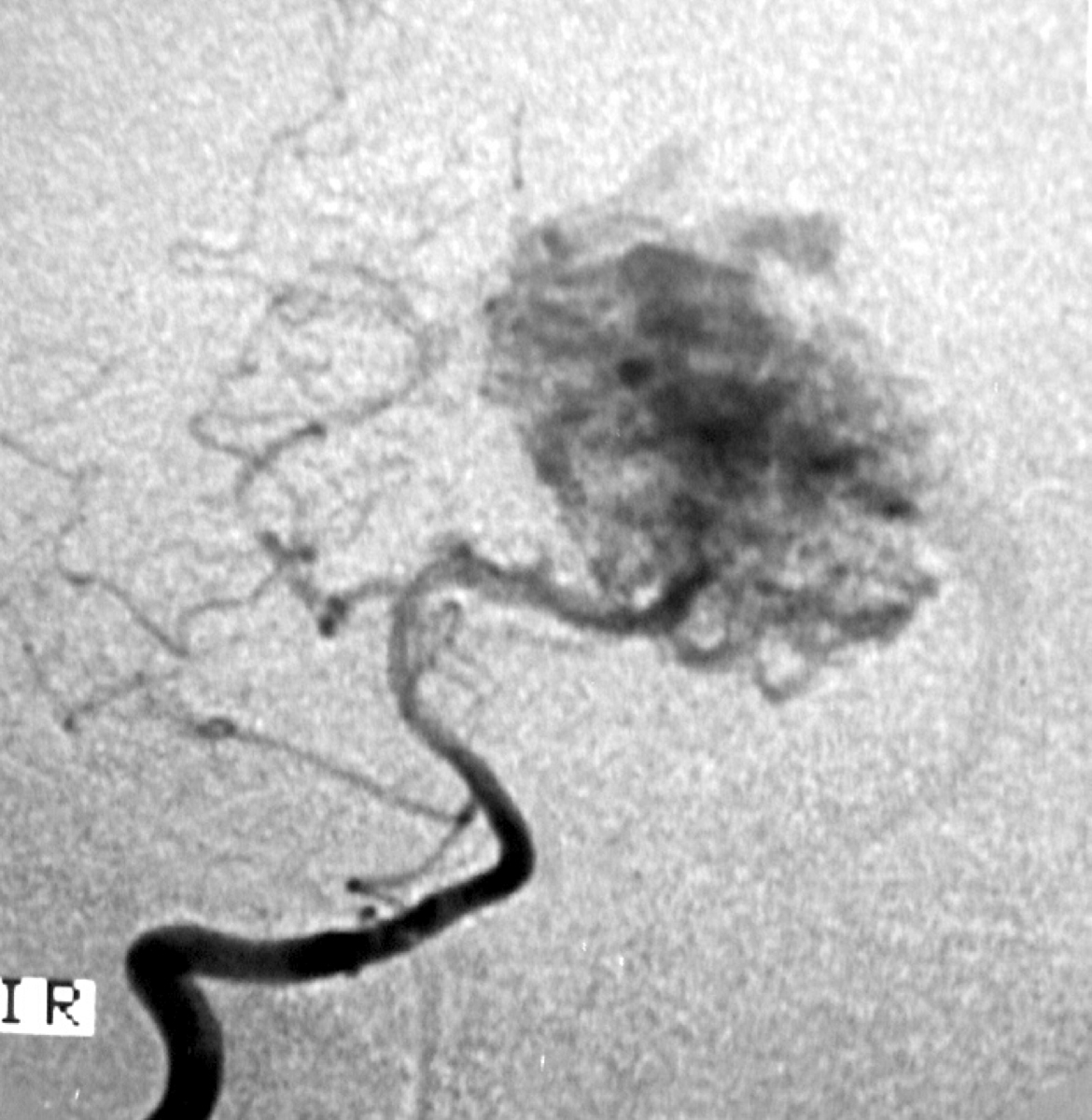


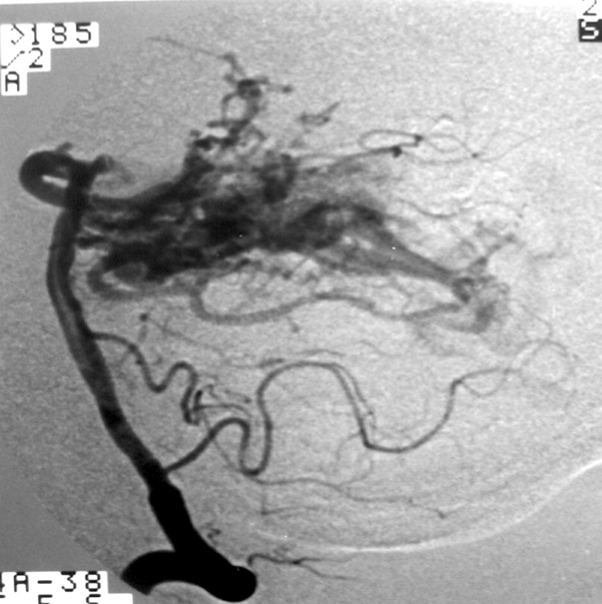
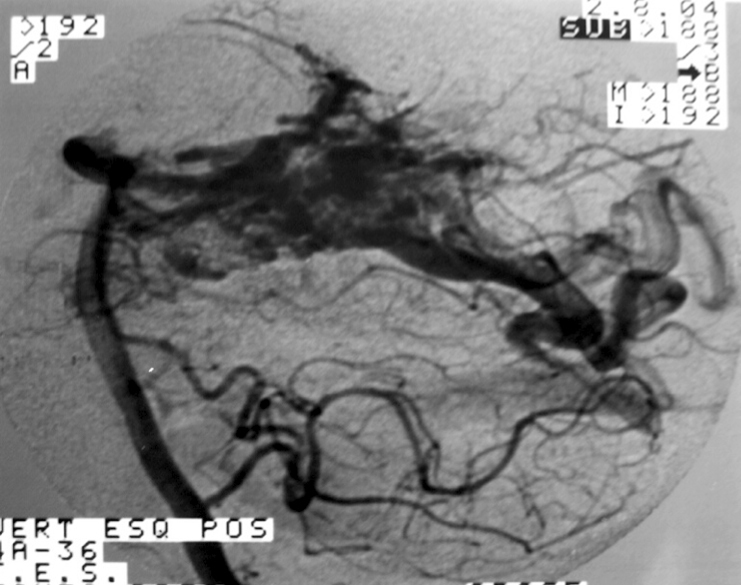
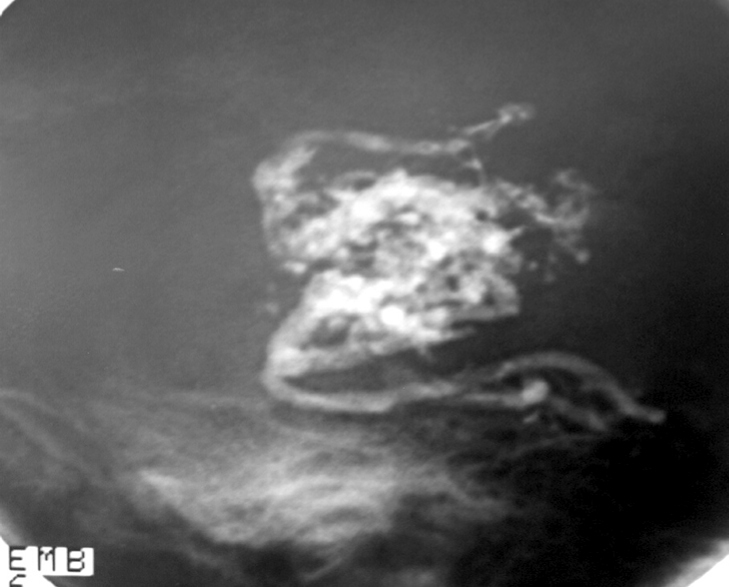
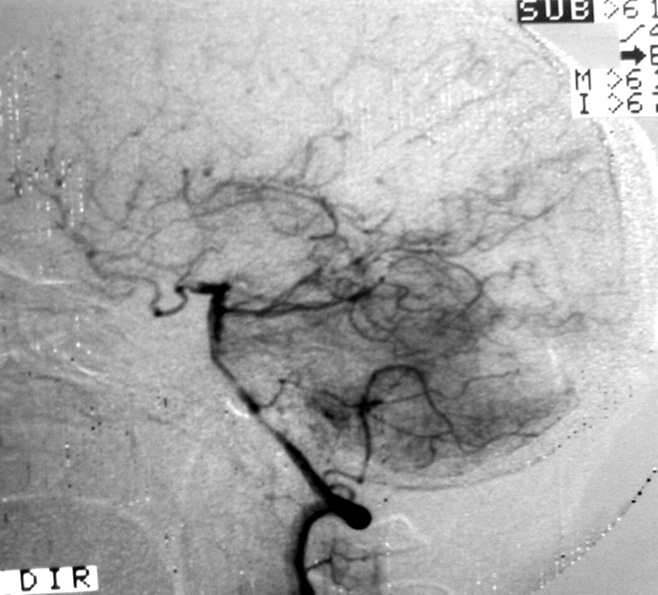

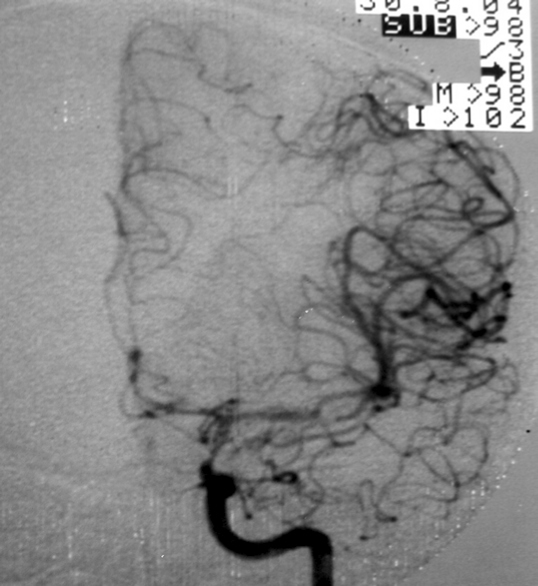
++++
3. Individualizing the treatment
Although these classifications are very useful as a general guide, these grading systems are very rigid and often raise doubts when deciding on a therapeutic decision. The ideal situation is to treat the patient in a reference center for vascular neurosurgery with a multidisciplinary team, formed by a vascular and endovascular neurosurgeons and a radiosurgeon to analyze each case carefully and chose the best option for the patient.
Several factors should be considered in this decision-making process :
– Team experience ;
– AVM size : one of the most important factors for any possible treatment. Large and complex AVMs, as SM grades 4 and 5, are hard to treat and are associated to high morbi-mortality rates [95]. Depending on the AVM, the treatment risk is as high as or even higher than its natural history [93] ;
– Location : mainly if close to eloquent areas ;
– Angioarchitecture : diffuse nidus, single draining vein, deep venous drainage mean greater challenges in the treatment ;
– Patient age : due to the 2 to 4% annual risk of bleeding, young patients should be treated whenever possible to minimize the risk of intracerebral hemorrhage in their lifetime ; [7]
– Clinical conditions : patients with relevant comorbidities are not good candidates for microsurgical resection, and thus less invasive options should be considered, such as embolization and radiosurgery ; [52]
– Presence of symptoms : duration and seriousness, as well as functional impact in the patient’s quality of life, should be evaluated. Complex AVMs (SM 4 and 5) with installed relevant neurological deficits may eventually be treated by surgical resection because this option will not be associated to new relevant deficits as it would be in cases of asymptomatic patients, besides also preventing new episodes of hemorrhage.
– Previous bleeding : although not necessarily related to risk of rebleeding in the long term, can mean a greater risk of rebleeding in the short and mid-terms ;
– Patient’s profession : because some post-treatment deficits can be very morbid depending on the activity.
++++
4. Therapeutic modalities
AVMs can be treated by microsurgical resection, endovascular embolization, or radiosurgery. Many times, it is necessary to use more than one modality of treatment to obtain the cure.
4.1. Surgical treatment
Surgical treatment has progressed considerably in recent years because of new microscopes, stereotactic guidance or neuronavigation, intraoperative electrophysiologic monitoring and intraoperative fluorescence video angiography using indocyanine green (ICG).
It is an elective procedure, when it presents with bleeding, surgery should usually be performed about two weeks after the ictus,that facilitates surgery. As observed by Lawton et al. [46] hematomas help separate AVMs from adjacent brain ; evacuation of hematoma creates working space around the AVM that can minimize transgression of normal brain or access a deep nidus that might otherwise have been unreachable ; and hemorrhage can obliterate some of the AVMs arterial supply to reduce its flow during resection. However when there is a voluminous intracerebral hematoma, causing intracranial hypertension that puts the life of the patient at risk, it is an emergency procedure. In such cases, an emergency drainage of the hematoma should be performed. However, if possible, a careful analysis based on CTA or DSA should take place in order to decide if it is possible to resect the nidus in the same surgery.
The complete excision of AVMs through microsurgery is still the treatment of choice in a great number of cases. (Figure 12)
Ideal AVMs to be treated by microsurgery are small (less than 3 cm in diameter) and symptomatic, located in non-eloquent areas.
Advantages are : immediate and permanent elimination of the risk of hemorrhage, improvement in neurological functions and reduction of seizure episodes [16, 34].
Disadvantages are : risks associated to craniotomy, general anesthesia, neurological deficits related to the surgery, and longer hospitalization when compared to other therapeutic options.
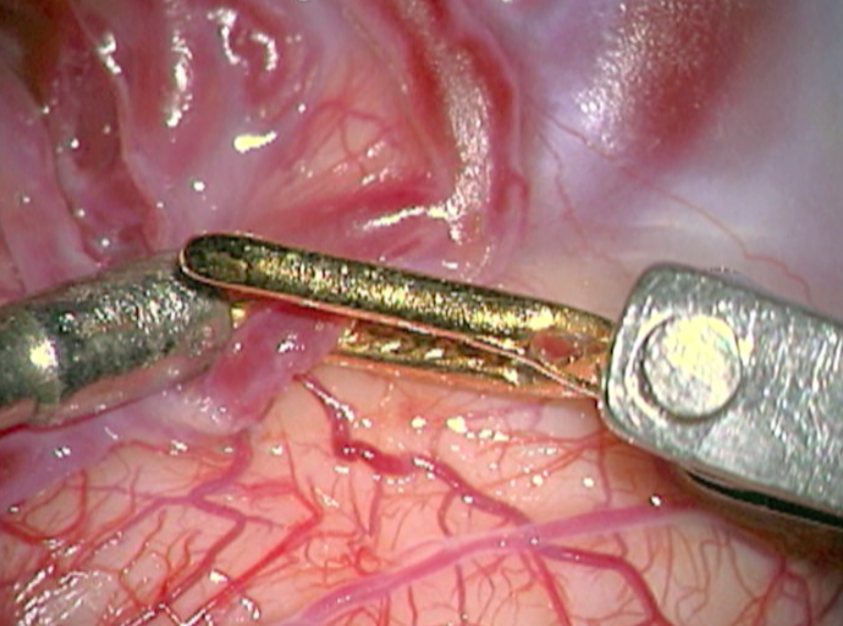
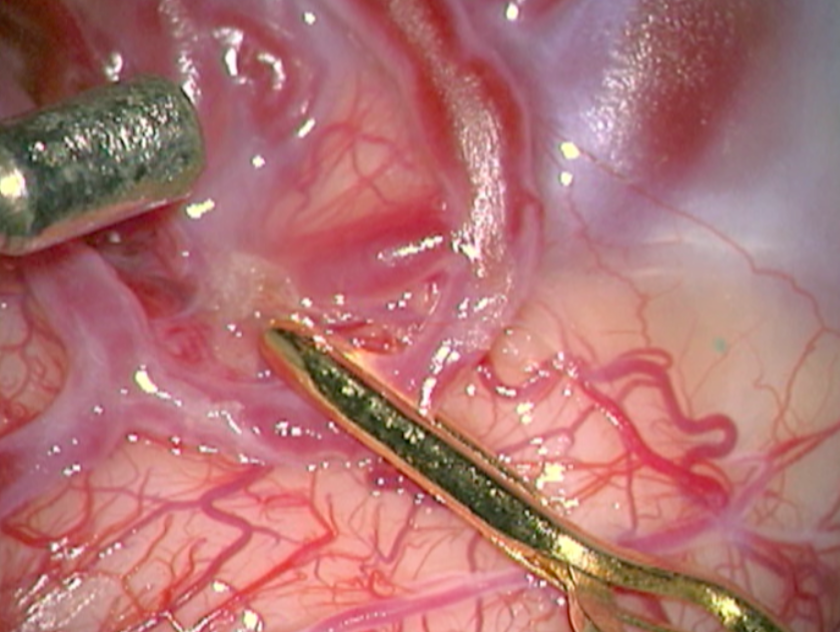
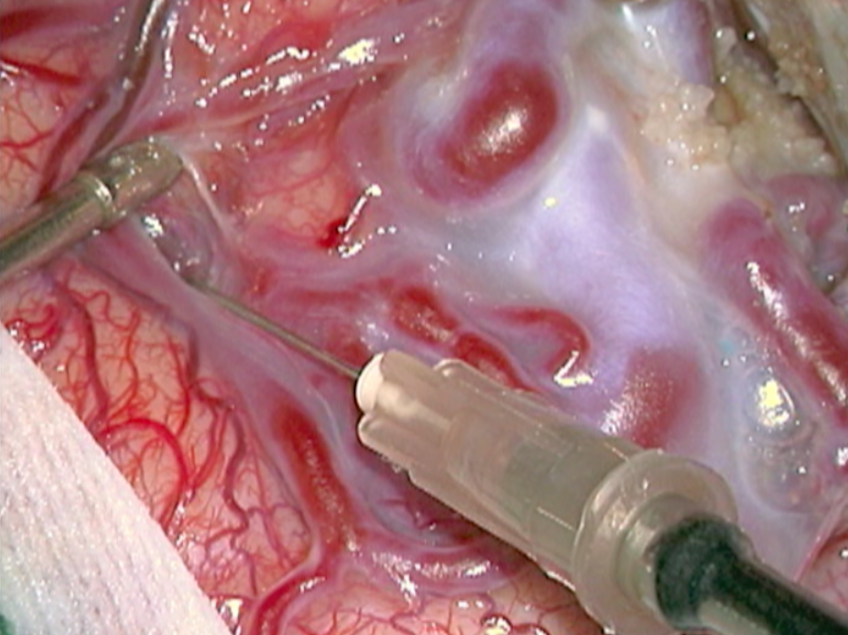
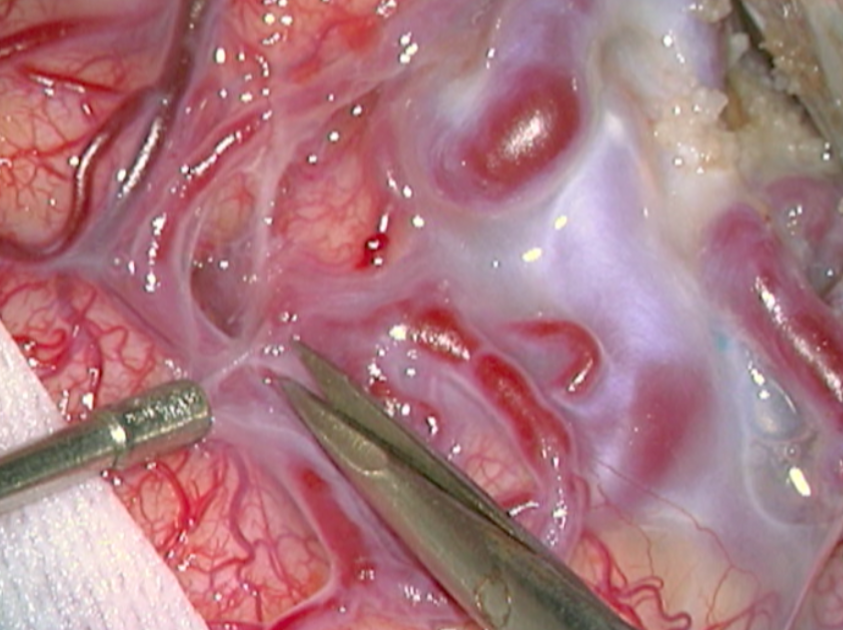

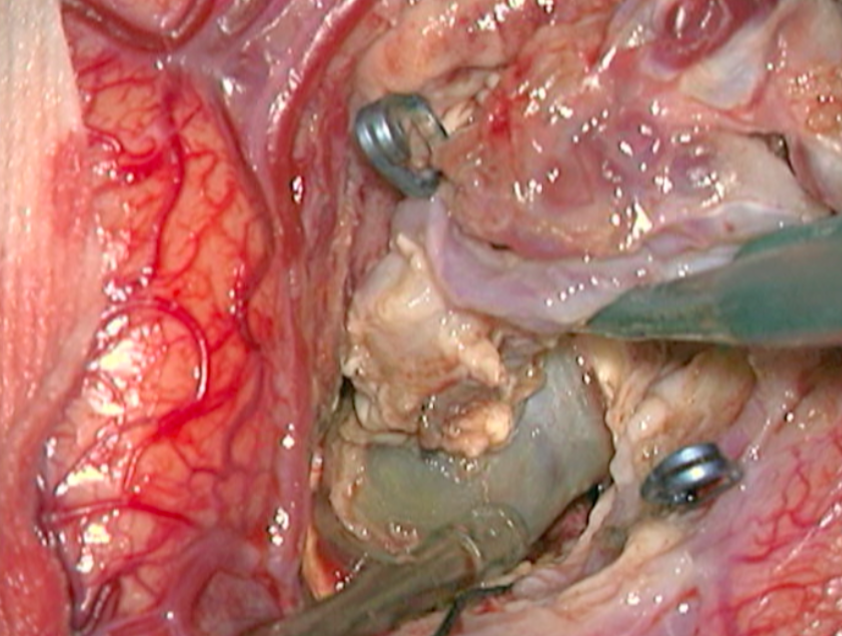
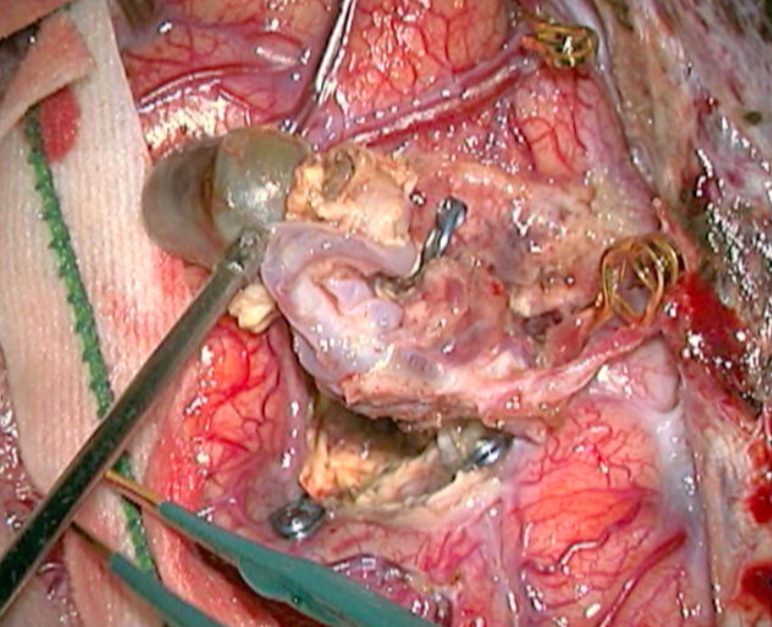
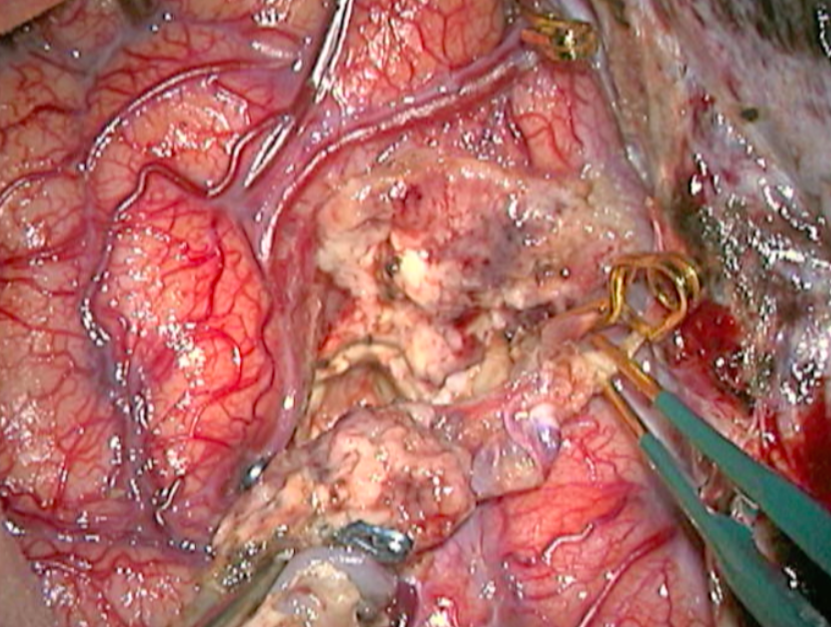
++++
4.2. Endovascular treatment
The endovascular approach can be meant to cure the AVM or to make microsurgery or radiosurgery easier. Small AVMs can be cured through embolization alone, but big or giant AVMs can rarely be completely eliminated through the endovascular method.
Embolization can be made with : n-Butyl cyanoacrylate (NBCA) ; polyvinyl alcohol (PVA) ; ethylene vinyl alcohol copolymer (Onyx).
Embolization indications :
– Patient prefers a non-invasive treatment and does not agree with neurosurgical intervention.
– Small AVMs with a single feeding artery : embolization alone, mainly when smaller than 1 cm [107].
– Cortical AVMs bigger than 3 cm in diameter : embolization should be considered to make microsurgery easier, by reducing the volume of the nidus, occluding arteriovenous fistulas and deep feeding arteries, thus facilitating the surgery.
– Prior to radiosurgery, in order to treat risk factors of bleeding such as intranidal aneurysms that may cause bleeding during the period radiosurgical takes to cure. Shrink the size of the AVM, particularly at the periphery, to reduce the size of the radiation field required for treatment. This indication must be analyzed judiciously, because the endovascular cast may deform the AVM limits, jeopardizing the radiosurgical planning.
Advantages are : immediate reduction of the AVM blood flow, thus greatly reducing the chance of the intraoperative phenomenon known as “normal perfusion pressure breakthrough” [97], besides the reduction of the surgical time and of blood loss [35].
Disadvantages are : although considered a minimally invasive procedure, complications happen in 6 to 14% of the cases, most are not serious. However some serious complications have been reported, such as intravascular adherence of the microcatheter, hemorrhage during or right after the embolization (in up to 5% of the cases) cerebral ischemia by erratic embolization, and death. [20, 107] Another disadvantage are the reduction of the AVM “compressibility” during surgery, the endovascular cast may deform the AVM limits to radiosurgical planning, and some times the deep feeders are not occluded.
It is important to always keep in mind that embolization is an invasive method that associates morbidity and mortality to the treatment, and that serial procedures lead to greater risks.
++++
4.3. Radiosurgical treatment (Figure 13 and Figure 14)
Stereotactic radiosurgery can be used alone or together with embolization. It may also be used to treat small residual nidus after microsurgery. It is indicated mainly for patients in poor clinical conditions or with small lesions, deep or near eloquent areas. The complete obliteration takes from 1 to 3 years after the procedure. Obliteration rates for small AVMs (< 3 cm) is relatively high (from 81 to 90%), [20, 39, 43, 102], but less than half of the bigger lesions are effectively cured through radiosurgery, for grade IV lesions obliterations of the order of 20% are reported. [66] The obliteration rate of AVMs smaller than 4 ml is reported to be 85–100%, larger than 4 mL is reported to be 40–58%, and when it is larger than 10 ml both non-obliteration and complication rates rise with only a 32% obliteration. [21, 53, 81] When the volume is greater than 15 ml the obliteration rate decrease to 25% over a 40month period. [81]
Radiosurgery indication :
– Patient prefer a non-invasive treatment and do not agree with neurosurgical intervention.
– High surgical risk : due to AVM location or poor clinical condition.
– Postoperative residual AVMs : mainly those located in deep cerebral areas.
– AVMs located in deep cerebral areas : diencephalon, basal ganglia, internal capsule, and brain stem.
Advantages are : avoids craniotomy and general anesthesia, allows the treatment of AVMs in deep locations or inaccessible with relatively low morbidity, low risk and no immediate discomfort.
Disadvantages are : complications vary and depend on the radiation dose. Headache, nausea and vomiting and seizures may occasionally happen. An important disadvantage is the time it takes for any curative effect to happen : two to three years ; and during this period the risk of hemorrhage remains as for non-treated AVMs. Therefore, 8-10% of the patients bleed while waiting for the therapeutic effect of radiation (bleeding rate of 4% per year). Symptomatic edema caused by the radiation (radionecrosis) is another relevant complication related to the location of the AVM and to higher radiation doses, happening in about 10% of the cases [19]. Radionecrosis may result in focal neurological deficit or seizures ; corticosteroids may help in the treatment, diminishing the sequel after several weeks or months. Transitory neurological worsening happens in approximately 5% of the cases and permanent neurological deficit may occur in 1.5 to 3% of the cases [57].
In the case of a large-volume AVM, radiosurgery (single dose) is associated with a significant risk of morbidity. Some strategies are used to reduce the risk of complication and try to increase the rate of obliteration as in staged-volume radiosurgery or hypofractionated stereotactic radiotherapy (also known as fractionated stereotactic radiosurgery).
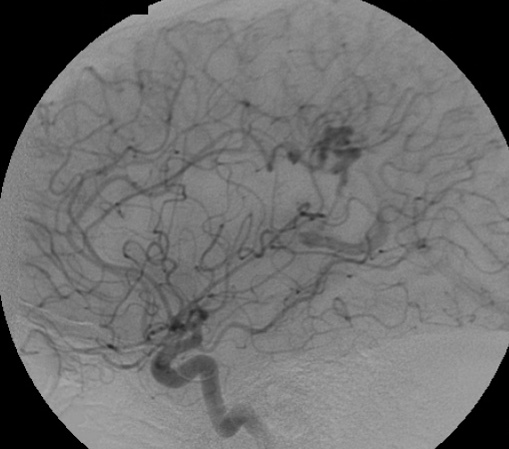
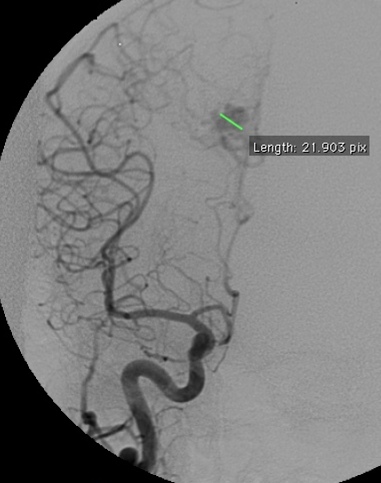
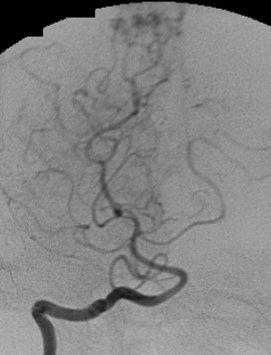
c) Angiography in an AP projection of the right vertebral artery showing an AVM fed by the right PCA.
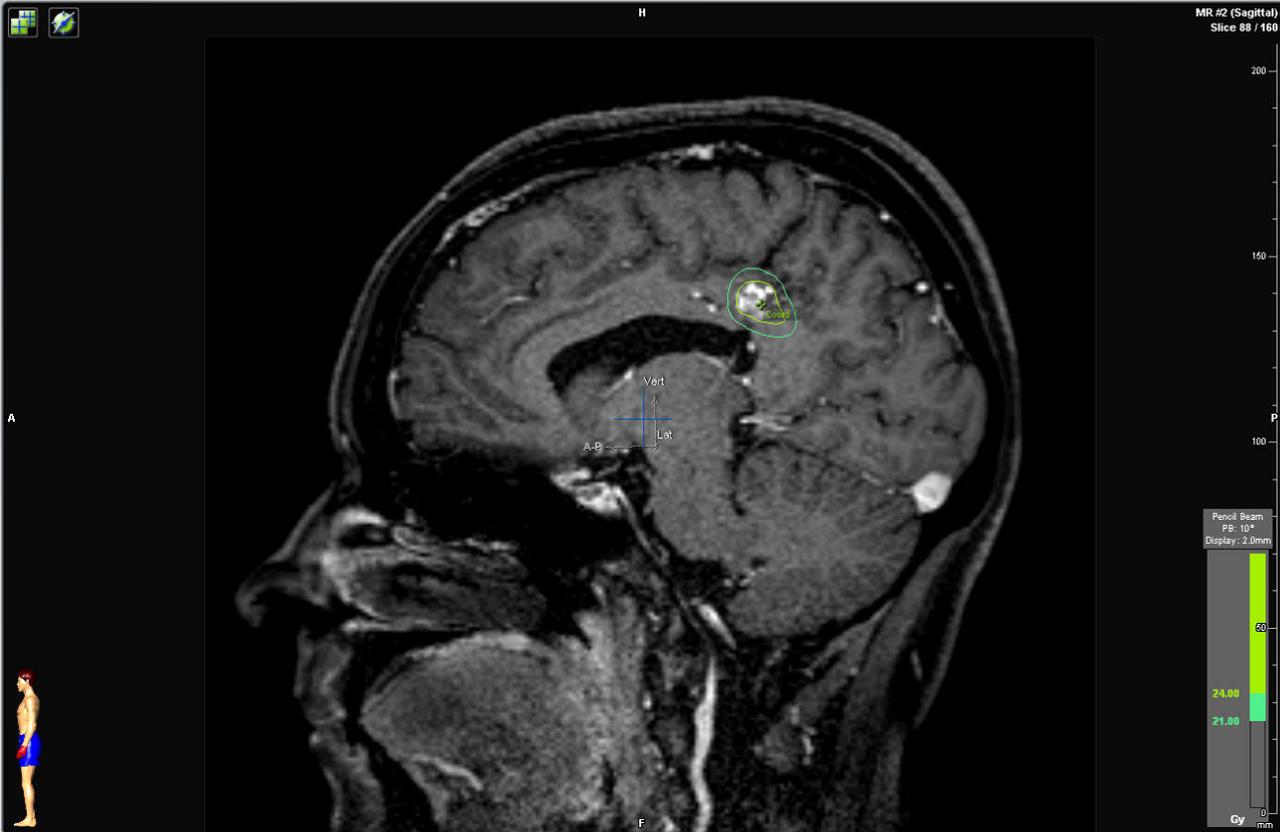
d) Sagittal cerebral T1-weighted MRI with contrast showing an AVM at the posterior aspect of the cingulum gyrus. Observe the radiosurgical planning with a prescription dose of 24 Gy.
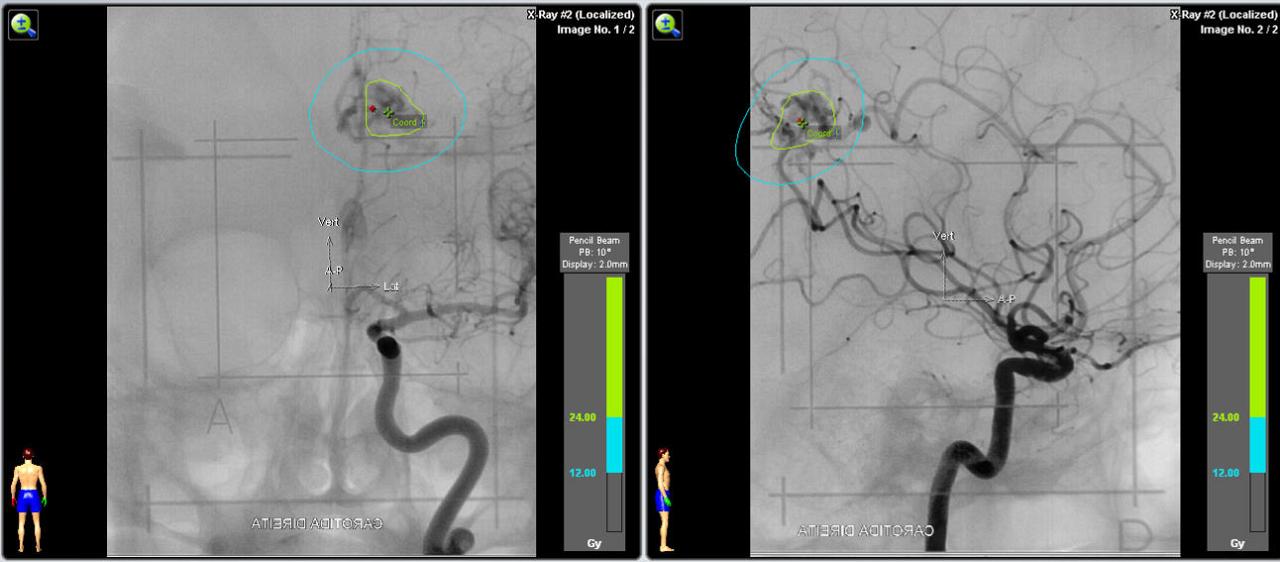
e) Angiography in an AP and lateral projection of the right internal carotid artery showing the AVM and the planning with a prescription dose of 24 Gy.
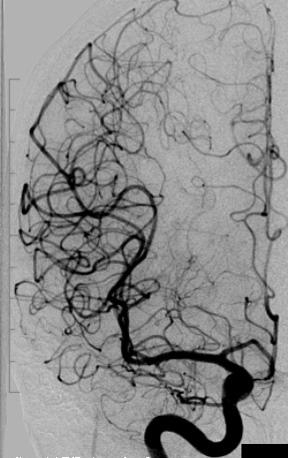
f) Two years postoperative angiography of the right internal carotid artery in an AP projection showing complete occlusion of the AVM.
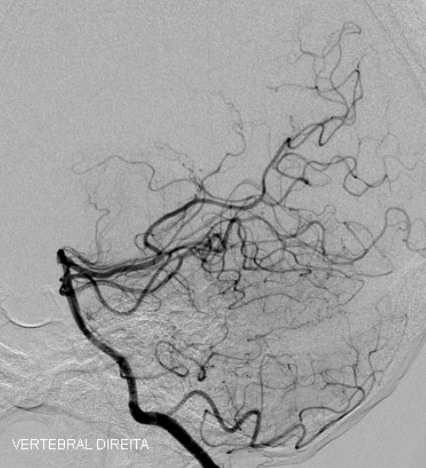
g) Two years postoperative angiography of the right vertebral artery in a lateral projection showing complete occlusion of the AVM.
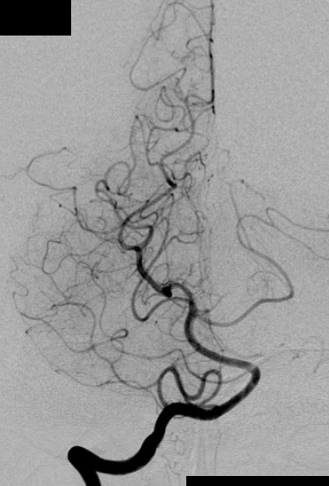
h) Two years postoperative angiography of the right vertebral artery in an AP projection showing complete occlusion of the AVM.

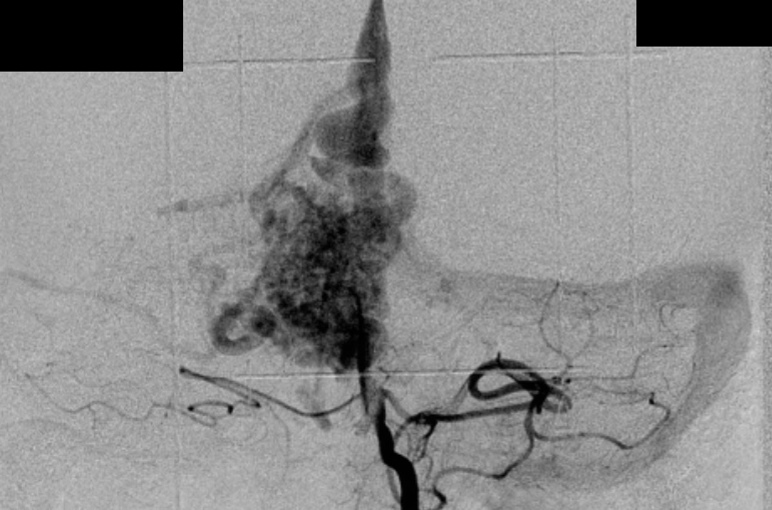
b) Angiography in an AP projection of the left vertebral artery showing an AVM fed by the PCA and superior cerebellar artery.
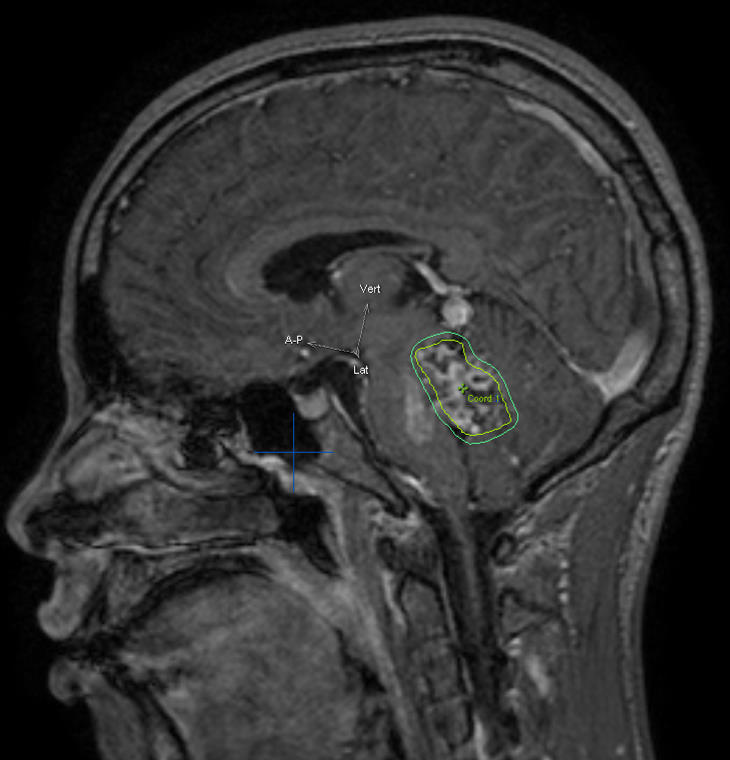
c) Sagittal cerebral T1-weighted MRI with contrast showing an AVM inside the fouth ventricle. Observe the radiosurgical planning.
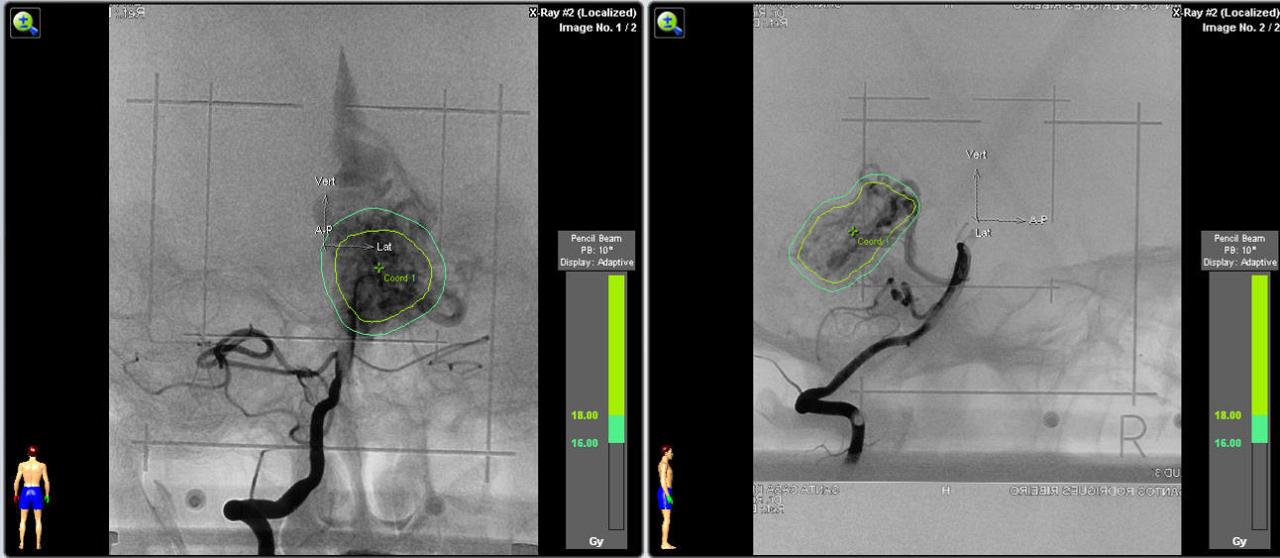
d) Angiography in an AP and lateral projection of the right internal carotid artery showing the AVM and the planning with a prescription dose of 18 Gy.
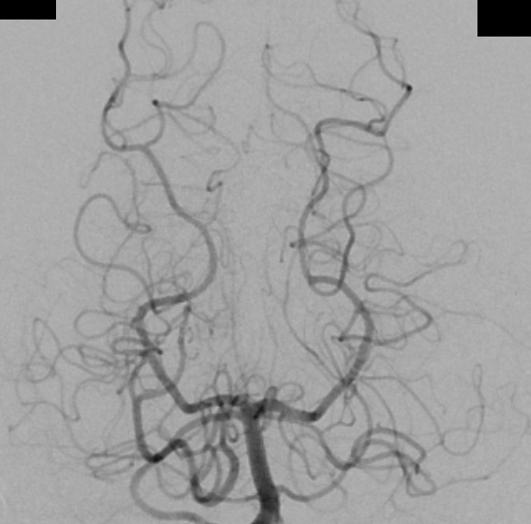
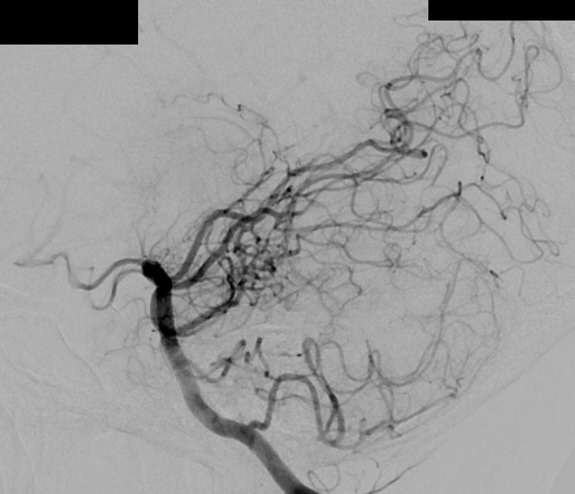
f) Two years postoperative angiography of the right vertebral artery in a lateral projection showing complete occlusion of the AVM.
++++
4.3.1. Staged Volume radiosurgery
Consists of dividing the AVM into equal volumetric portions and treating each one as a different radiosurgical section, with intervals of 6 to 9 months between staged treatments. Some authors advocate that the basal portions must be treated before more superficial portions, the most medial portion before more lateral portions and portions of the AVM filled in carotid before portions filled in vertebral artery angiography. [3, 11] Major draining veins should be irradiated last to minimize the risk of obstruction of venous outflow.
Staged radiosurgery is a viable and relatively safe treatment for large AVMs, in otherwise untreatable patients. [3, 11, 86, 91] Chung et al. [11] in a series of six patients (volumes treated ranged from 47 to 72 cm3) with a mean total follow-up of 28 months (range from 15 to 54) total obliteration was reported in 33.3% and incomplete obliteration 66.7% ; no patient developed radiation-related clinically complications. Amponsah et al. [3] in a series of five patients (volumes treated ranged from 22 to 50 cm3) with a mean total follow-up of 81.2 months (range from 42 to 120 months) reported total obliteration in 40%, 40% near total obliteration and 20% poor obliteration ; radiation-related mild complication were observed in 40% of patients.
4.3.2. Hypofractionated stereotactic radiotherapy (HFSRT)
It should be offered only to patients with AVMs unsuitable to surgery or single fraction procedure because of relatively low probability of obliteration after the fractionated irradiation. It still remains an attractive option for patients with otherwise untreatable AVMs that present repeated hemorrhage, progressive neurological deficits, intractable seizures, and other severe symptoms. [105]
The AVM is completely irradiated in multiple sections (3 to 6) with low dose (4 to 7 Gy, the total doses usually ranged from 28 to 42 Gy). [9, 105] However, the results are below expectation if compared with the results of stereotactic radiosurgery for selected, small lesions. The treatment protocols, radiotherapy devices, mean AVM size and characteristics, and follow up are very heterogeneous in literature, and so are the rates of obliteration and complications. Blamek et al. [9] reported a rate of total obliterated of only 21% and an additional 34% of partial obliterations after three years of follow-up. Xiao et al. [110] reported no lesion obliterated, however, they observed a significant decrease in the volume of patent AVMs and propose that this may turn some of these AVMs into manageable lesions with a single-dose of SRS or microsurgery. Lindvall et al. [51] observed better results, with obliteration rates of 56% and 81% for lesions of volume 4-10 cm3 and from 50% to 70% for lesions larger than 10 cm3 after two and five years of follow-up, respectively.
The complication rate varies in literature from low rates of radiological changes and mild symptoms to 86% radiographic changes and 28% symptomatic. [104, 105]
In the first years after treatment, the annual risk of bleeding is about 2% (similar to no treated patient). The HFSRT does not increase the risk of subsequent hemorrhage. [9, 110]
++++
VIII. Complications
Morbidity, mortality and obliteration rates following treatment of AVMs vary significantly in literature according to specific AVM characteristics. Generally, surgical mortality of approximately 3% and a permanent morbidity of 9%, embolization related complications of roughly 10%, and morbidity after radiosurgery of approximately 10% have been reported [27, 28, 83]
There are many complications related to the treatment of AVMs : bleeding, ischemic stroke, venous thrombosis, vasospasm, infection, seizure, radionecrosis (radiosurgery), etc.
Although infrequent, there is a complication that deserves more comment, because it is potentially devastating and specific to post intracranial high flow AVM treatment (resection, occlusion or radiosurgery), that is the post-operative brain swelling and hemorrhage [115]. There are two hypothesis to explain this phenomenon : the normal perfusion pressure breakthrough (NPPB) and occlusive hyperemia (OH).
++++
1. Normal Perfusion Pressure Breakthrough
First described in 1978 by Spetzler e al. [97], the hypothesis suggests that the parenchyma surrounding a high-flow AVM is chronically hypoperfused ; as a result, it has impaired autoregulation, rendering it vulnerable to the normal perfusion pressure likely to be seen following AVM resection.
Thus, following removal of an AVM, the local capillary beds and arterioles in the remaining normal parenchyma experience increased perfusion but lack the ability to vasoconstrict and auto regulate. This could lead, in some cases, to hyperemia, compromise of the capillary beds, and resultant edema and/or hemorrhage.
++++
2. Occlusive Hyperemia
In 1993, Al-Rodhan et al. [1] proposed this alternative explanation for postoperative hemorrhage and edema following AVM resection and gave some evidence against the NPPB hypothesis. They conclude that “ the complications of hemorrhage and edema associated with the resection of high-flow AVM’s are the result of two separate but interrelated mechanisms involving both the arterial feeders and the venous drainage systems. 1) Stagnant arterial flow in former AVM feeders and their parenchymal branches with subsequent worsening of the existing hypoperfusion and ischemia and subsequent hemorrhage and/or edema into these areas, and ; 2) obstruction of the venous outflow system of the brain adjacent to the AVM with subsequent passive hyperemia, engorgement, and further arterial stagnation. In addition, disturbed autoregulation at the microcirculation level may play a role.”
The precise pathophysiology mechanism of the hemorrhage and edema that can manifest after AVM resection is not completely understood. In literature there is a lot of evidence in favor of and against these two hypotheses. As Zacharia et al. [115] we believe that ultimately these hypotheses are not mutually exclusive and perhaps exist in a spectrum of hemodynamic alteration following AVM resection.
++++
IX. Follow-up
Postoperative DSA is essential to confirm the complete resection of the AVM. If the DSA confirms the complete resection, the AVM rarely reoccurs, except in pediatric patients. For those patients, the DSA must be repeated five years after the initial treatment.
The natural history of AVMs partially treated or residual is the same for lesions that are not treated ; therefore the partial treatment is rarely justified. Residual or recurrent AVMs need to be evaluated for further treatment, and the risk should be individually analyzed.
++++
Bibliography
- Al-Rodhan NR, Sundt TM Jr, Piepgras DG, et al. Occlusive hyperemia : a theory for the hemodynamic complications following resection of intracerebral arteriovenous malformations. J Neurosurg 1993 ; 78:167. C4
- Al-Shahi R, Bhattacharya JJ, Currie DG, Papanastassiou V, Ritchie V, Roberts RC, Sellar RJ, Warlow CP. Prospective, population-based detection of intracranial vascular malformations in adults : the Scottish Intracranial Vascular Malformation Study (SIVMS). Stroke. 2003 ; 34:1163–1169. C4
- Amponsah K, Ellis TL, Chan MD, Bourland JD, Glazier SS, McMullen KP, Shaw EG, Tatter SB. Staged Gamma Knife Radiosurgery for Large Cerebral Arteriovenous Malformations. Stereotact Funct Neurosurg 2011 ; 89:365–371. C4
- Andrade-Souza YM, Zadeh G, Ramani M, Scora D, Tsao MN, Schwartz ML. Testing the radiosurgery- based arteriovenous malformation score and the modified Spetzler-Martin grading system to predict radiosurgical outcome. J Neurosurg 2005 ; 103:642–648. C4
- ApSimon HT, Reef H, Phadke RV, Popovic EA. A population-based study of brain arteriovenous malformation : long-term treatment outcomes. Stroke 2002 ; 33:2794-2800. C4
- Batjer H, Suss RA, Samson D. Intracranial arteriovenous malformations associated with aneurysms. Neurosurgery 1986 ; 18:29-35. C4
- Batjer HH, Devous MD Sr, Seibert GB, Purdy PD, Bonte FJ. Intracranial arteriovenous malformation : relationship between clinical factors and surgical complications. Neurosurgery 1989 ; 24:75-79. C4
- Berman MF, Sciacca RR, Pile-Spellman J, Stapf C, Connolly ES Jr, Mohr JP, Young WL. The epidemiology of brain arteriovenous malformations. Neurosurgery 2000 ; 47:389-397. C4
- Blamek S, Larysz D, Miszczyk L, Idasiak A, Rudnik A, Tarnawski R. Hypofractionated stereotactic radiotherapy for large or involving critical organs cerebral arteriovenous malformations. Radiol Oncol 2013 ; 47(1):50-56. C4
- Brown RD Jr, Wiebers DO, Torner JC, O’Fallon WM. Incidence and prevalence of intracranial vascular malformations in Olmsted County, Minnesota, 1965 to 1992. Neurology 1996 ; 46:949-952. C4
- Chung WY, Shiau CY, Wu SM, Liu KD, Guo WY, Wang LW, Pan DH. Staged radiosurgery for extra-large cerebral arteriovenous malformations : method, implementation, and results. J Neurosurg 2008 ; 109:65-72. C4
- Cockroft KM, Jayaraman MV, Amin-Hanjani S, Derdeyn CP, McDougall CG, Wilson JA. A Perfect Storm : How A Randomized Trial of Unruptured Brain Arteriovenous Malformations’ (ARUBA’s) Trial Design Challenges Notions of External Validity. Stroke. 2012 ; 43:1979-1981. C4
- Colby GP, Coon AL, Huang J, Tamargo RJ. Historical Perspective of Treatments of Cranial Arteriovenous Malformations and Dural Arteriovenous Fistulas. Neurosurg Clin N Am 2012 ; 23:15–25. C4
- Courville CB. Vascular anomalies of the brain, in Pathology of the central nervous system : a study based upon a survey of lesions found in a series of forty thousand autopsies. Mountain View, Pacific Press, 1950 ; ed 3:142-152. C4
- Crawford PM, West CR, Chadwick DW, et al. Arterio-venous malformations of the brain : natural history in unoperated patients. J Neurol Neurosurg Psychiatry 1986 ; 49(1):1–10. C4
- Drake CG : Cerebral arteriovenous malformations : considerations for and experience with surgical treatment in 166 cases. Clin Neurosurg 1979 ; 26:145-208. C4
- Du R, Hashimoto T, Tihan T, Young WL, Perry V, Lawton MT. Growth and regression of arteriovenous malformations in a patient with hereditary hemorrhagic telangiectasia. Case report. J Neurosurg. 2007 ; 106 : 470–477. C4
- Fink GR. Effects of cerebral angiomas on perifocal and remote tissue : a multivariate positron emission tomography study. Stroke 1992 ; 23:1099-1105. C4
- Flickinger JC, Kondziolka D, Maitz AH, Lunsford LD : Analysis of neurological sequelae from radiosurgery of arteriovenous malformations : how location affects outcome. Int J Radiat Oncol Biol Phys 1998 ; 40:273-278. C4
- Friedlander RM. Clinical Practice. Arteriovenous Malformations of the Brain. N Engl J Med 2007 ; 356:2704-2712. C4
- Friedman WA, Bova FJ, Mendenhall WM. Linear accelerator radiosurgery for arteriovenous malformations : The relationship of size to outcome. J Neurosurg. 1995 ; 82:180–189. C4
- Gallione CJ, Richards JA, Letteboer TG, Rushlow D, Prigoda NL, Leedom TP, Ganguly A, Castells A, Ploos van Amstel JK, Westermann CJ, Pyeritz RE, Marchuk DA. SMAD4 mutations found in unselected HHT patients. J Med Genet. 2006 ; 43:793–797. C4
- Graf CJ, Perret GE, Torner JC : Bleeding from cerebral arteriovenous malformations as part of their natural history. J Neurosurg 1983 ; 58:331-337. C4
- Gross CR, Kase CS, Mohr JP, Cunningham SC, Baker WE. Stroke in south Alabama : incidence and diagnostic features – a population-based study. Stroke 1984 ; 15:249-255. C4
- Hamilton MG, Spetzler RF : The prospective application of a grading system for arteriovenous malformations. Neurosurgery 1994 ; 34:2–7. C2
- Hartmann A, Mast H, Mohr JP, Koennecke HC, Osipov A, Pile-Spellman J, Duong DH, Young WL. Morbidity of intracranial hemorrhage in patients with cerebral arteriovenous malformation. Stroke 1998 ; 29:931-934. C2
- Hartmann A, Mast H, Mohr JP, Pile-Spellman J, Connolly ES, Sciacca RR, Khaw A, Stapf C. Determinants of staged endovascular and surgical treatment outcome of brain arteriovenous malformations. Stroke. 2005 ; 36(11):2431-2435. B2
- Hartmann A, Stapf C, Hofmeister C, Mohr JP, Sciacca RR, Stein BM, Faulstich A, Mast H. Determinants of neurological outcome after surgery for brain arteriovenous malformation. Stroke. 2000 ; 31 (10):2361-2364. B2
- Hashimoto N, Nozaki K. Do cerebral arteriovenous malformations recur after angiographically confirmed total extirpation ? Crit Ver Neurosurg 1999 ; 9:141-146. C4
- Hashimoto N, Nozaki K, Takagi Y, et al. Surgery of cerebral arteriovenous malformations. Neurosurgery 2007 ; 61(Suppl 1):375–87. C4
- Hernesniemi JA, Dashti R, Juvela S, et al. Natural history of brain arteriovenous
malformations : a long-term follow-up study of risk of hemorrhage in 238 patients. Neurosurgery 2008 ; 63(5):823–829. C4 - Heros RC, Korosue K, Diebold PM : Surgical excision of cerebral arteriovenous malformations : Late results. Neurosurgery 1990 ; 26:570–578. C4
- Hillman J. Population-based analysis of arteriovenous malformation treatment. J Neurosurg 2001 ; 95 : 633-637. C4
- Houser OW, Campbell JK, Campbell RJ, Sundt TM, Jr. : Arteriovenous malformation affecting the transverse dural venous sinus—an acquired lesion. Mayo Clin Proc 1979 ; 54:651-661.C4
- Jafar JJ, Davis AJ, Berenstein A, Choi IS, Kupersmith MJ : The effect of embolization with N-butyl cyanoacrylate prior to surgical resection of cerebral arteriovenous malformations. J Neurosurg 1993 ; 78:60-69. C4
- Jane JA, Kassell NF, Torner JC, et al. The natural history of aneurysms and arteriovenous malformations. J Neurosurg 1985 ; 62(3):321–323. C4
- Jellinger K. Vascular malformations of the central nervous system : a morphological overview. Neurosurg Rev 1986 ; 9:177-216. C4
- Jessurun GAJ, Kamphuis DJ, van der Zande FHR, Nossent JC. Cerebral arteriovenous malformations in the Netherlands Antilles : high prevalence of hereditary hemorrhagic telangiectasia-related single and multiple cerebral arteriovenous malformations. Clin Neurol Neurosurg 1993 ; 95:193-198. C4
- Karlsson B, Kihlstrom L, Lindquist C, Steiner L : Gamma knife surgery for previously irradiated arteriovenous malformations. Neurosurgery 1998 ; 42:1-5. C4
- Kasliwal MK, Kale SS, Gupta A, Kiran NAS, Sharma MS, Sharma BS, et al. Outcome after hemorrhage following Gamma Knife surgery for cerebral arterivenous malformations. Clinical article. K Neurosurg 2009 ; 110:1003-1009. C4
- Kihlström L, Guo WY, Karlsson B, et al : Magnetic resonance imaging of obliterated arteriovenous malformations up to 23 years after radiosurgery. J Neurosurg 1997 ; 86:589–593. C4
- Kikuchi K, Kowada M, Sasajima H. Vascular malformations of the brain in hereditary hemorrhagic telangiectasia (Rendu-Osler-Weber disease). Surg Neurol 1994 ; 41:374-380. C4
- Kondziolka D, Lunsford LD, Flickinger JC : Gamma knife stereotactic radiosurgery for cerebral vascular malformations, in Alexander E, Loeffler JS, Lunsford LD (eds) : Stereotactic Radiosurgery. New York, McGraw-Hill, 1993 ; 147-155. C4
- Laufer L, Cohen A. Sturge-Weber syndrome associated with a large left hemispheric arteriovenous malformation. Pediatr Radiol 1994 ; 24:272-273. C4
- Lawton MT. UCSF Brain Arteriovenous Malformation Study Project. Spetzler-Martin Grade III arteriovenous malformations : surgical results and a modification of the grading scale. Neurosurgery 2003 ; 52(4):740-748. C4
- Lawton MT, Du R, Tran M, et al. Effect of presenting hemorrhage on outcome after microsurgical resection of brain arteriovenous malformations. Neurosurgery 2005 ; 56(3):485–493.90. C4
- Lawton MT, Kim H, McCulloch CE, Mikhak B, Young WL. A supplementary grading scale for selecting patients with brain arteriovenous malformations for surgery. Neurosurgery 2010 ; 66(4):702–713. C4
- Leblanc GG, Golanov E, Awad IA, Young WL, Biology of Vascular Malformations of the Brain NINDS Workshop Collaborators. Biology of vascular malformations of the brain. Stroke 2009 ; 40(12):694-702. C4
- Lee J, Girvigian MR, Miller M, Rahimian J, Chen J, Greathouse H, Tome M. Validation of a radiosurgery- based grading system for arteriovenous malformations. Radiosurgery 2006 ; 6:221–228. C4
- Lehecka M, Laakso A, Hernesniemi J. Helsinki Microneurosurgery Basics and Tricks. Edition 2011. C4
- Lindvall P, Bergström P, Löfroth PO, Hariz MI, Henriksson R, Jonasson P, et al. Hypofractionated conformal stereotactic radiotherapy for arteriovenous malformations. Neurosurgery 2003 ; 53 : 1036-1042. C4
- Luessenhop AJ, Gennarelli TA. Anatomical grading of supratentorial arteriovenous malformations for determining operability. Neurosurgery 1977 ; 1:30-35. C4
- Lunsford LD, Kondziolka D, Flickinger JC, Bissonette DJ, Jungreis CA, Maitz AH, et al. Stereotactic radiosurgery for arteriovenous malformations of the brain. J Neurosurg. 1991 ; 75:512–524. C4
- Manchola IF, De Salles AA, Foo TK, Ackerman TH, Candia GT, Kjellberg RN. Arteriovenous malformation hemodynamics : a transcranial Doppler study. Neurosurgery 1993 ; 33 : 556-562. C4
- Marchuk DA, Srinivasan S, Squire TL, Zawistowski JS. Vascular morphogenesis : tales of two syndromes. Hum Mol Genet.2003 ;12:R97–R112 C4
- Marks MP, Lane B, Steinberg GK, Chang P. Vascular characteristics of intracranial arteriovenous malformations in patients with clinical steal. AJNR Am J Neuroradiol 1991 ; 12:489-496. C4
- Maruyama K, Kawahara N, Shin M, et al. The risk of hemorrhage after radiosurgery for cerebral arteriovenous malformations. N Engl J Med 2005 ; 352:146-153. C4
- Mast H, Mohr JP, Osipov A, Pile-Spellman J, Marshall RS, Lazar RM, Stein BM, Young WL. “Steal” is an unestablished mechanism for the clinical presentation of cerebral arteriovenous malformations. Stroke 1995 ; 26:1215-1220. C4
- Mast H, Young WL, Koennecke H-C, Sciacca RR, Osipov A, Pile-Spellman J, Hacein-Bey L, Duong H, Stein BM, Mohr JP. Risk of spontaneous hemorrhage after diagnosis of cerebral arteriovenous malformation. Lancet 1997 ; 350:1065-1068. C4
- McCormick WF. Classification, pathology, and natural history of angiomas of the central nervous system. Wkly Update Neurol Neurosurg 1978 ; 14:2-7. C4
- McCormick WF. Pathology of vascular malformations of the brain, in Wilson CB, Stein BM (eds) : Intracranial arteriovenous malformations. Baltimore, Williams & Wilkins, 1984 ; 44-63. C4
- McCormick WF. The pathology of vascular ("arteriovenous") malformations. J Neurosurg. 1966 ; 24(4):807-816.C3
- McCormick WF, Boulter TR. Vascular malformations ("angiomas") of the dura mater. J Neurosurg. 1966 ; 25(3):309-311. C4
- McCormick WF, Nofzinger JD. "Cryptic" vascular malformations of the central nervous system. J Neurosurg. 1966 ; 24(5):865-75. C4
- Minakawa T, Tanaka R, Koike T, Takeuchi S, Sasaki O. Angiographic follow-up study of cerebral arteriovenous malformations with reference to their enlargement and regression. Neurosurgery. 1989 ; 24:68–74. C4
- Miyawaki L, Dowd C, Wara W, Goldsmith B, Albright N, Gutin P, Halbach V, Hieshima G, Higashida R, Lulu B, Pitts L, Schell M, Smith V, Weaver K, Wilson C, Larson D. Five year results of LINAC radiosurgery for arteriovenous malformations : outcome for large AVMs. Int J Radiat Oncol Biol Phys 1999 ; 44:1089–1106. C4
- Moftakhar P, Hauptman JS, Malkasian D, Martin NA. Cerebral arteriovenous malformations. Part 1 : cellular and molecular biology. Neurosurg Focus 2009 ; 26 (5):E10. C4
- Moftakhar P, Hauptman JS, Malkasian D, Martin NA. Cerebral arteriovenous malformation. Part 2 : physiology. Neurosurg Focus 2009 ; 26 (5):E11. C4
- Mohr JP, Moskowitz AJ, Parides M, Stapf C, Young WL. Hull Down on the Horizon : A Randomized Trial of Unruptured Brain Arteriovenous Malformations (ARUBA) Trial. Stroke. 2012 ; 43:1744-1745. C4
- Mohr JP, Moskowitz AJ, Stapf C, Hartmann A, Lord K, Marshall SM, et al. The ARUBA trial : current status, future hopes. Stroke. 2010 ; 41:537–540. C4
- Mohr JP, Parides MK, Stapf C, Moquete E, Moy CS, Overbey JR, Al-Shahi Salman R, Vicaut E, Young WL, Houdart E, Cordonnier C, Stefani MA, Hartmann A, von Kummer R, Biondi A, Berkefeld J, Klijn CJ, Harkness K, Libman R, Barreau X, Moskowitz AJ ; international ARUBA investigators. Medical management with or without interventional therapy for unruptured brain arteriovenous malformations(ARUBA) : a multicentre, non-blinded, randomized trial. Lancet 2014 ; 383(9917):614-621. B1
- Mokin M, Dumont TM, Levy,EI. Novel Multimodality Imaging Techniques for Diagnosis and Evaluation of Arteriovenous Malformations. Neurol Clin 2014 ; 32:225–236. C4
- Moussa R, Harb A, Menassa L, Risk T, Nohra G, Samaha E, Mohasseb G, Okais N, Awad I. Etiologic spectrum of intracerebral hemorrhage in young patients. Neurochirurgie. 2006 ; 52:105-109. C4
- Nataf F, Meder JF, Roux FX, Blustajn J, Merienne L, Merland JJ, et AC. Angioarchitecture associated with haemorrhage in cerebral arteriovenous malformations : a prognostic statistical model. Neuroradiology 1997 ; 39:52-58. C4
- Newton TH, Cronqvist ST. Involvement of dural arteries in intra-cranial arteriovenous malformations. Radiology 1969 ; 93:1071—1078. C4
- Nogueira RG, Bayrlee A, Hirsch JA, Yoo AJ, Copen WA. Dynamic contrast-enhanced MRA at 1.5 T for detection of arteriovenous shunting before and after Onyxembolization of cerebral arteriovenous malformations. J Neuroimaging. 2013 ; 23(4):514-517. C4
- Norris JS, Valiante TA, Wallace MC, Willinsky RA, Montanera WJ, terBrugge KG, et al. A simple relatioship between radiological arteriovenous malformation hemodynamics and clinical presentation : a prospective, blinded analysis of 31 cases. Neurosurg 1999 ; 90:673–679. C4
- Ogilvy CS, Stieg PE, Awad I, et al. AHA Scientific Statement : recommendations for the management of intracranial arteriovenousmalformations : a statement for healthcare professionals from a special writing group of the Stroke Council, American Stroke Association. Stroke. 2001 ; 32:1458-1471. C4
- Ondra SL, Troupp H, George ED, Schwab K : The natural history of symptomatic arteriovenous malformations of the brain : a 24-year follow-up assessment. J Neurosurg 1990 ; 73:387-391. C4
- Orrison WW Jr, Snyder KV, Hopkins LN, et al. Whole-brain dynamic CT angiography and perfusion imaging. Clin Radiol 2011 ; 66:566–574. C4
- Pan DH, Guo WY, Chung WY, Shiau CY, Chang YC, Wang LW. Gamma knife radiosurgery as a single treatment modality for large cerebral arteriovenous malformations. J Neurosurg. 2000 ; 93(Suppl 3):113–119. C4
- Pawlikowska L, Tran MN, Achrol AS, Ha C, Burchard E, Choudhry S, Zaroff J, Lawton MT, Castro R, McCulloch CE, Marchuk D, Kwok PY, Young WL. Polymorphisms in transforming growth factor-beta-related genesALK1 and ENG are associated with sporadic brain arteriovenous malformations. Stroke. 2005 ; 36:2278–2280. B3
- Pollock BE, Brown RDJ. Use of the Modified Rankin Scale to assess outcome after arteriovenous malformation radiosurgery. Neurology. 2006 ; 67(9):1630-1634. C4
- Pollock BE, Flickinger JC. A proposed radiosurgery-based grading system for arteriovenous malformations. J Neurosurg 2002 ; 96:79–85. C4
- Pollock BE, Flickinger JC. Modification of the radiosurgery-based arteriovenous malformation grading system. Neurosurgery 2008 ; 63(2):239-243. C4
- Pollock BE, Kline RW, Stafford SL, Foote RL, Schomberg PJ : The rationale and technique of staged-volume arteriovenous malformation radiosugery. Int J Radiat Oncol Biol Phys 2000 ; 48:817–824. C4
- Redekop G, TerBrugge K, Montanera W, Willinsky R : Arterial aneurysms associated with cerebral arteriovenous malformations : classification, incidence, and risk of hemorrhage. J Neurosurg 1984 ; 89:539-546. C4
- Saleh RS, Lohan DG, Villablanca JP, et al. Assessment of craniospinal arteriovenous malformations at 3T with highly temporally and highly spatially resolved contrast-enhanced MR angiography. AJNR Am J Neuroradiol 2008 ; 29:1024-1031. C3
- Samson D, Batjer HH. Preoperative evaluation of the risk/benefit ratio for arteriorvenous malformations of the brain. In : Wilkins RH, Rengachary SS, editors. Neurosurgery Update II. New York : McGraw-Hill ; 1991. p. 121–33 C4
- Sato S, Kodama N, Sasaki T, Matsumoto M, Ishikawa T. Perinidal dilated capillary networks in cerebral arteriovenous malformations. Neurosurgery 2004 ; 54:163-170. C4
- Sirin S, Kondziolka D, Nirajan A, Flickinger JC, Maitz AH, Lunford LD : Prospective staged volume radiosurgery for large arteriovenous malformations : indications and out-comes in otherwise untreatable patients. Neurosurgery 2006 ; 58:17–27. C4
- Sisti MB, Kader A, Stein BM : Microsurgery for 67 intracranial arteriovenous malformations less than 3 cm in diameter. J Neurosurg 1993 ; 79 : 653–660. C4
- Spears J, Terbrugge KG, Moosavian M, Montanera W, Willinsky RA, Wallace MC, et al. A discriminative prediction model of neurological outcome for patients undergoing surgery of brain arteriovenous malformations. Stroke 2006 ; 37:1457-1464. C4
- Spetzler RF, Hargraves RW, McCormick PW, Zarbramski JM, Flom RA, Zimmerman RS. Relatioship of perfusion pressure and size to risk hemorrhage from arteriovenous malformations. J Neurosurg 1992 ; 76:918–923. C4
- Spetzler RF, Martin NA : A proposed grading system for arteriovenous malformations. J Neurosurg 1986 ; 65:476-483. C4
- Spetzler RF, Ponce FA. A 3-tier classification of cerebral arteriovenous malformations. J Neurosurg 2011 ; 114:842–849. C4
- Spetzler RF, Wilson CB, Weinstein P, Mehdorn M, Townsend J, Telles D. Normal perfusion pressure breakthrough theory. Clin Neurosurg 1978 ; 25:651-672. C4
- Stapf C, Mast H, Sciacca RR, Berenstein A, Nelson PK, Gobin YP, Pile-Spellman J, Mohr JP ; New York Islands AVM Study Collaborators. The New York Islands AVM Study : design, study progress, and initial results. Stroke 2003 ; 34(5):e29-33. C2
- Stapf C, Mast H, Sciacca RR, Choi JH, Khaw AV, Connolly ES, Pile-Spellman J, Mohr JP. Predictors of hemorrhage in patients with untreated brain arteriovenous malformation. Neurology 2006 ; 66:1350-1355. C4
- Stapf C, Moskowitz AJ, Parides MK, et al. Abstract 189 : ARUBA—a Randomised trial of Untruptured brains AVMs. Stroke. 2013 ;44:A189. C4
- Starke RM, Komotar RJ, Connolly ES. A randomized trial of unruptured brain arteriovenous malformations. Neurosurgery 2013 ; 73(4):13-15. C4
- Steinberg GK, Chang SD, Levy RP, Marks MP, Frankel K, Marcellus M : Surgical resection of large incompletely treated intracranial arteriovenous malformations following stereotactic radiosurgery. J Neurosurg 1996 ; 84:920-928. C4
- The Arteriovenous Malformation Study Group. Arteriovenous Malformations of the Brain in Adults. N Engl J Med 1999 ; 340:1812-1818. C4
- Veznedaroglu E, Andrews DW, Benitez RP, Downes MB, Werner-Wasik M, Rosenstock J, et al. Fractionated stereotactic radiotherapy for the treatment of large arteriovenous malformations with or without previous partial embolization. Neurosurgery. 2004 ; 55:519–530. C4
- Wang HC, Chang RJ, Xiao F. Hypofractionated stereotactic radiotherapy for large arteriovenous malformations. Surg Neurol Int. 2012 ; 3(Suppl 2):105–110. C4
- Weber W, Kis B, Siekmann R, Jans P, Laumer R, Kühne D. Preoperative embolization of intracranial arteriovenous malformations with Onyx. Neurosurgery 2007 ; 61:244-254. C4
- Weber W, Kis B, Siekmann R, Kuehne D. Endovascular treatment of intracranial arteriovenous malformations with onyx : technical aspects. AJNR Am J Neuroradiol 2007 ; 28:371-377. C4
- Wilkins RH : Natural history of intracranial vascular malformations : a review. Neurosurgery 1985 ; 16:421-430. C4
- Wilson CB. Cryptic vascular malformations. Clin Neurosurg 1992 ; 38:49-84. C4
- Xiao F, Gorgulho AA, Lin CS, Chen CH, Agazaryan N, Viñuela F, et al. Treatment of giant cerebral arteriovenous malformation : hypofractionated stereotactic radiation as the first stage. Neurosurgery 2010 ; 67 : 1253-1259 C4
- Yamamoto M, Jimbo M, Hara M, et al : Gamma knife radio-surgery for arteriovenous malformations : long-term follow-up results focusing on complications occurring more than 5 years after irradiation. Neurosurgery 1996 ; 38:906–914. C4
- Yasargil MG (1987) Pathologic considerations. In : Yasargil MG (ed) Microneurosurgery, ACM of the brain : history, embryology, pathologic considerations, hemodynamics, diagnosis studies, microsurgical anatomy, vol 3A, Thieme, New York, pp 3-22. C4
- Yasargil MG (1987) Pathologic considerations. In : Yasargil MG (ed) Microneurosurgery, ACM of the brain : history, embryology, pathologic considerations, hemodynamics, diagnosis studies, microsurgical anatomy, vol 3A, Thieme, New York, pp 49-212. C4
- Zabel-du Bois A, Milker-Zabel S, Huber P, Schlegel W, Debus J : Stereotactic LINAC- based radiosurgery in the treatment of cerebral arteriovenous malformations located deep, involving corpus callosum, motor cortex, or brainstem. Int J Radiat Oncol Biol Phys 2006 ; 64:1044–1048. C4
- Zacharia BE, Bruce S, Appelboom G, Connolly ES Jr. Occlusive hyperemia versus normal perfusion pressure breakthrough after treatment of cranial arteriovenous malformations. Neurosurg Clin N Am. 2012 ; 23(1):147-51. C4